This article is about gas properties (chemical and physical), which are need to know in liquefaction process. Gas Carriers carry gas in a liquefied form. This is the liquid state of substances that under “normal” conditions would be gaseous. Most liquefied gases are hydrocarbons, which is the collective name for a group of organic substances that are made up of molecules of hydrogen and carbon.
- Introduction
- Liquefied Petroleum Gases (LPG)
- Liquefied Natural Gas (LNG)
- Liquefied Chemical Gases
- Chemical Properties of the Gases
- Alkanes
- Alkenes and Alkynes
- Physical Properties of the Gases
- States of Matter
- Gases – Properties and Rules
- First Stage – Gas to Liquid
- Gases and Vapours
- Saturated Vapour Pressure
- Liquid and Vapour Densities
- Effect of Pressure on Containment
- Refrigeration
- Lubrication and Viscosity
- Temperature Conversion
- What are the Gases Carried on Liquefied Gas Carriers Used For?
Introduction
Understanding the nature and behaviour of hydrocarbons, both in liquid and gaseous form, is critical to their safe carriage. This article will describe in detail the important characteristics and their implications.
Liquefied Gas Carrier hull types | Type “A”, “B” and “C” tanks | Are known as independent tank types, they are completely self-supporting and do not form part of the ship’s hull. Three types of independent tank are allowed, depending upon the design pressure. |
---|---|---|
![]() | Type “A” Tanks | Are constructed from low temperature steel (Arctic D) of a prismatic shape that makes use of the maximum space within the hull. The maximum allowable vapour pressure in this type of system is normally 0,25 kg/cm2. This means that cargoes must be carried in a fully refrigerated condition, at or near atmospheric pressure. To ensure safety in the event of cargo tank leakage, a secondary containment system is required to protect the ship’s hull from low temperatures. This secondary containment system is known as a “secondary barrier” and is a feature of all ships with Type “A” tanks that are capable of carrying cargoes at temperatures of below -10 °C. |
![]() | Type “B” Tanks | Are generally spheres, although provision is made for constructing these tanks of plane surfaces. This type of containment system is subjected to a thorough stress analysis and must include fatigue life and crack propagation analysis. Because of these design factors, the Type “B” tank requires only a partial secondary barrier comprising of a drip tray and a splash barrier. A protective steel dome covers the primary barrier above deck level, and insulation is applied to the outside of the primary barrier surface. The spherical Type “B” tank was originally designed for LNG carriage, but can also be used in LPG carriers (normally ethylene). |
![]() | Type “C” Tanks | Are used in semi and fully-pressurised gas carriers and is the construction used for deck tanks on all LPG carriers. These tanks are designed and built to conventional pressure vessel codes and, as a result, are subject to accurate stress analysis. Where this type of system is used, no secondary barrier is required and the hold space (when located within the vessel’s hull) can be filled with either inert gas or air. In the case of a typical fully-pressurised ship (ambient temperature carriage), the tank may be designed for 17 kg/cm2. |
![]() | Membrane Tank Type | Is based on having a very thin primary barrier or “membrane” that is supported by a layer of insulation within the confines of the hull of the ship. The membrane system differs from the self-supporting tanks in that the membrane containment system must be provided with a complete secondary barrier to ensure cargo integrity in the event of leakage of the primary barrier. There are two principal types of membrane system in common use, both named after the companies who developed them, Gaz Transport and Technigaz (these companies have now merged to create Gaz Transport Technigaz GTT). |
The International Maritime Organization (IMO), for the purposes of its Gas Carrier Codes, defines liquefied gases carried at sea as:
“Liquids with a vapour pressure exceeding 2,8 bar absolute at a temperature of 37,8 °C.”
Pressured measured at 37,8 °C are known as Reid Vapour Pressures.
The primary objective of this article is to ensure that you understand the full meaning, chemistry and implications of that definition.
Liquefied gases are divided into three categories:
- LPG – or Liquefied Petroleum Gases.
- LNG – or Liquefied Natural Gases.
- Chemical Gases.
Liquefied Petroleum Gases (LPG)
LPG covers propane, butane or butane/propane mixtures.
The two main sources of LPG are:
- From processing the wet natural gas that is retrieved from gas or oil fields. Both LPG and Natural Gas Liquids are removed from the natural gas in this manner.
- By fractionation of crude oil at petroleum refineries or natural gasoline manufacturing plants. LPG is one of the by-products of the crude oil distillation process. Before shipping, LPG must be purified by removing any sour sulphur compounds and then drying the gas.
Liquefied Natural Gas (LNG)
LPG is a naturally occurring mixture of hydrocarbons that are liquefied under high pressure and at a low temperature. Its composition is between 80 % to 99 % methane and 1 % to 17 % ethane, the balance comprising a mixture of propane, butane, pentane and nitrogen.
Liquefied Chemical Gases
Tanker Safety TrainingThis category will also discuss chemical gases that possess similar physical properties to LPG and that are transported in bulk by gas carriers.
The most important of these “chemical” products are:
- anhydrous ammonia,
- ethylene,
- vinyl chloride,
- butadiene.
These chemical gases are “intermediate products” and are subject to additional processing before they are delivered for final use.
Chemical Properties of the Gases
Hydrocarbons are compounds of carbon and hydrogen and are amongst the most important organic compounds.
“An organic substance is not the same as a “natural” substance. In chemical terms it means that it contains carbon.”
All hydrocarbons contain a carbon “backbone”, which may be straight, branched or even form a ring. The hydrogen atoms are attached to that backbone in varying numbers.
The simplest example of a hydrocarbon is methane, which is naturally occurring and sometimes referred to as swamp or marsh gas. Methane will be referred to by its molecular formula, CH4. This means that each carbon atom has 4 atoms of hydrogen attached to it.
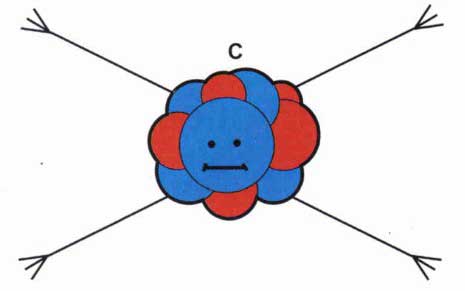
Ethane, whose molecular formula is C2H4, is another naturally occurring hydrocarbon. Its formula tells us that it has 2 atoms of carbon and 4 atoms of hydrogen.
Methane and ethane are both constituents of the gases that are trapped above the oil in most oil wells.
It is important to understand the importance of the physical properties of methane, ethane and a third hydrocarbon, known as ethyne or acetylene (C2H2).
Read also: Cargo Containment Systems of LPG and LNG
Many chemical compounds, and especially hydrocarbons, can exist in different geometric configurations. Some compounds can have the same molecular formula but be linked together in different ways. A structural formula allows us to show the arrangements of atoms in a way that a chemical formula cannot. This is important because understanding the structure of the molecule tells us how strong the links that bind the compound are. The stronger the links, the more stable the compound.
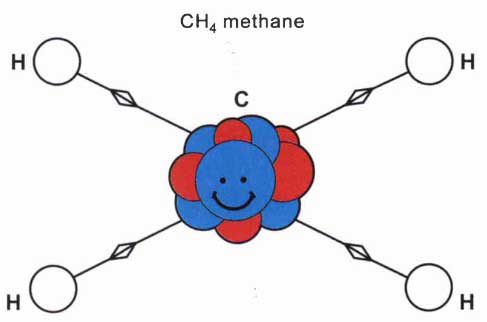
Holding a Hydrogen Atom
An important concept before we begin to look at the structure is the “valence”, also known as valency or valency number which is a measure of the number of chemical bonds (arms) formed by the atoms of an element.
A good way to think of this is to imagine the carbon atom as an item with 4 “arms”, Which would make its valency number “4”. At all times, each of the arms must to be holding on to something. By preference it will hold onto the arm of another atom that is different to itself. But when there’s no alternative, it will hold onto another like itself.
Because it is part bonded to another carbon atom, the preference to hold onto something different to itself will always make a structure like the one in picture 3 less “stable”.

Other in Addition to Hydrogen Atoms
So the CH4 structure is very stable and will stay that way in most pressure and temperature conditions.
The number of bonds formed by a given element was originally thought to be a fixed chemical property. Although this is not actually the case, we are lucky that for virtually all of their compounds, carbon forms four bonds and hydrogen one, so this is a rule/assumption we are able to safely follow.
To put all of this into practice and understand the implications for carriage on board ship, with our understanding of bonding and stability we can now immediately make some assumptions about the relative stability of the 3 structural formulae here, even if at this stage we know nothing else about them at all.
Alkanes
Methane is the first member of the series of hydrocarbon compounds where all valencies (arms) are used to the greatest combining power. Each of these compounds is said to be saturated and they are known as Alkanes. They are sometimes given the alternative name of “Paraffins”.
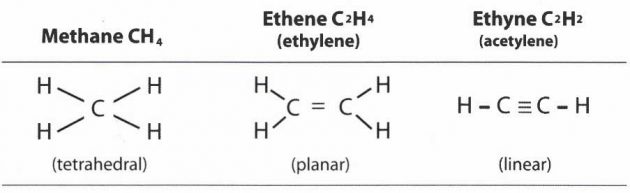
Alkanes generally show a lower reactivity because their C-H and C-C bonds are relatively stable and cannot be easily broken. Alkanes are both important raw materials of the chemical industry and also the most important fuels of the world economy.
The general molecular formula of an Alkane is:
CnH(2n+2)
where:
- n – is the number of atoms.
Name of Compound | Formula | State at STP |
---|---|---|
Methane | CH4 | Gas |
Ethane | C2H6 | Gas |
Propane | C3H8 | Gas |
Butane | C4H10 | Gas |
Pentanes | C5H12 | Liquid |
Hexanes | C6H14 | Liquid |
The atoms in alkanes with four or more carbon atoms can be arranged in multiple ways to form different isomers, which are molecules that contain the same number and kind of atoms but have a different structure. “Normal” alkanes have a linear, unbranched configuration. (see the structural formulae for n-butane and iso-butane below).
“Isomers are molecules with the same chemical formula and often with the same kinds of bonds between them.”
To differentiate between the isomers, the linear unbranched isomer is usually referred to as the normal compound (and so has the prefix “n” when the compound is named) and the branched chain one is known as the “iso” compound.
To use butane as an example, here are its 2 structural formulae:
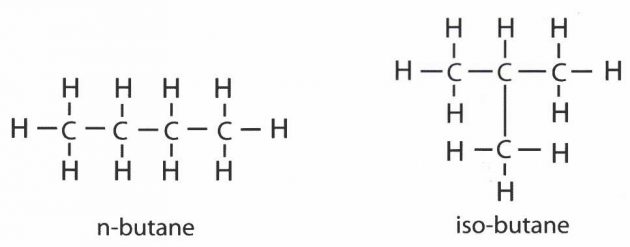
If we count up the carbon and hydrogen atoms, we will see that they both contain the expected 4 atoms of carbon and 10 of hydrogen. Their formulae will be written as follows:
n-butane CH3 ⋅ CH2 ⋅ CH2 ⋅ CH3
iso-butane CH3 ⋅ HC2H3 ⋅ CH3 or (CH3)2 ⋅ CH ⋅ CH3
The number of isomers increases rapidly with the number of carbon atoms; for alkanes with 1 to 12 carbon atoms, the number of isomers equals 1, 1, 1, 2, 3, 5, 9, 18, 35, 75, 159, and 355.
“Make sure you understand why this is written like this before you carry on.”
Methane and ethane are the main components of natural gas; they are normally stored as gases under pressure. However, it is easier to transport them as liquids, although this requires both compression and cooling of the gas. Propane and butane can be liquefied at fairly low pressures, and are well known as
liquefied petroleum gas (LPG).
The most important sources of alkanes are natural gas and oil. Natural gas primarily contains methane and ethane, with some propane and butane. Oil is a mixture of liquid alkanes and other hydrocarbons. Both were formed when dead marine animals and plants sank to the bottom of ancient seas, were covered with sediments in an oxygen-poor environment and then converted over many millions of years at high temperatures and high pressure to their current form.
Methane is constantly re-formed in large quantities, but the liquid and solid rarely develop to a considerable extent in nature and the present deposits will not be re-formed once they are exhausted. Solid alkanes (tar) occur as evaporation residues from oil.
All of the gases are flammable and will burn in air and/or oxygen to produce heat, carbon dioxide and water vapour. Because they are relatively non-reactive they do not present chemical compatibility problems with the materials commonly used in handling although, in the presence of moisture, the saturated hydrocarbons may form hydrates.
Because saturated hydrocarbons are colourless and odourless in their natural state, a harmless, non-toxic chemical called a mercaptan is added to make it easier to detect. Mercaptans contain sulphur and in a concentrated form the smell is almost unbearable.
“A mercaptan is a stench additive.”
It takes only a few parts per million to give natural gas a smell and the process is referred to as “stenching”.
Alkenes and Alkynes
Where double bonds occur between 2 carbon atoms, such as in the ethene (ethylene) example, and where triple bonds occur, such as in ethyne (acetylene), the carbon is called “unsaturated”. These structures belong to hydrocarbon alkene and alkyne groups.
Alkenes
Alkenes are sometimes referred to as olefins.
The general formula for alkenes is CnH2n. The first stable member of the series is ethylene or ethene (C2H4). The names of the members of the olefin series end in -ene (instead of -ane for the Alkanes) and indicate double bonds between the carbon atoms.
The physical properties of alkenes are comparable with alkanes. The physical state depends on molecular mass and the simplest alkenes, such as ethylene, propylene and butylenes, are gases at STP Linear alkenes of approximately five to sixteen carbons are liquids, and higher alkenes are waxy solids.
Alkenes are relatively stable compounds, but are more reactive than alkanes.
Alkynes
The alkynes are traditionally known as acetylenes, although the name acetylene is also used to refer to the simplest member of the series, known officially as ethyne.
The general formula for alkynes is CnHn.
Unlike alkanes, alkynes are unstable and very reactive. This gives rise to the intense heat (>3000 °C) of the acetylene flame in conjunction with oxygen as oxyacetylene, which is used for cutting steel.
Physical Properties of the Gases
In the previous section it was shown that the physical properties of a gas are dependent on its molecular structure and that while some compounds may have the same simple molecular formula, the ways in which the atoms are arranged may be quite different.
The examples of n-butane and iso-butane showed how compounds may have the same relative molecular mass but still have different physical (and chemical) properties.
However, there are other factors that determine the behaviour and properties of a gas or liquefied gas. These are temperature and pressure.
The principal physical properties of liquefied gases are listed at Table 1. It is important to note the different values of boiling point, liquid relative density and other physical constants, as the properties shown in Table 1 identify much of the care necessary when handling these cargoes.
Table 1. Physical Properties of Liquefied Gases | ||||||
---|---|---|---|---|---|---|
Liquefied Gas | Boiling Point (°C) | Critical Temperature (°C) | Critical Pressure (kg/cm2) | Condensing Ratio (dm3/Nm3) | Liquid Relative Density (H20=1) | STP Vapour Relative Density (Air=1) STP |
Methane | -161,5 | -82,5 | 45,6 | 0,804 | 0,474 | 0,554 |
Ethane | -88,6 | 32,1 | 49,8 | 2,453 | 0,548 | 1,048 |
Propane | -42,3 | 96,8 | 43,4 | 3,38 | 0,583 | 1,55 |
n-Butane | -0,5 | 153 | 38,8 | 4,32 | 0,603 | 2,09 |
i-Butane | -11,7 | 133,7 | 38,9 | 4,36 | 0,596 | 2,07 |
Ethylene | -103,9 | 9,90 | 51,5 | 2,20 | 0,570 | 0,975 |
Propylene | -47,7 | 92,1 | 46,5 | 3,08 | 0,613 | 1,48 |
α-Butylene | -6,1 | 146,4 | 39,7 | 4,01 | 0,624 | 1,94 |
γ-Butylene | -6,9 | 144,7 | 39,5 | 4,00 | 0,627 | 1,94 |
Butadiene | -5,0 | 161,8 | 44,0 | 3,81 | 0,647 | 1,88 |
VCM | -13,8 | 158,4 | 53,9 | 2,87 | 0,965 | 2,15 |
Ethylene Oxide | 10,73 | 195,7 | 75,8 | 2,13 | 0,896 | 1,52 |
Propylene Oxide | 34,2 | 209,1 | 48,6 | 0,830 | 2,00 | |
Ammonia | -33,4 | 132,4 | 115,2 | 1,12 | 0,683 | 0,597 |
Chlorine | -34 | 144 | 78,6 | 2,03 | 1,56 | 2,49 |
The single most important physical property of a liquefied gas is its saturated vapour pressure.
“You should review this table again once you have completed the article. lf the relevance of each of the columns is not clear to you at that point, you should re-read this chapter or seek further assistance.”
States of Matter
To understand how the gases become liquefied and the impact of this on containment and handling, we need to go back to some basic definitions and concepts.
Gas, liquid, and solid are the three states of matter or material. A solid has a definite shape and volume. A liquid has a definite volume but it takes the shape of a container whereas a gas fills the entire volume of a container.
Most substances can exist in either a solid, liquid or vapour state. There are specific terms used for each of the changes from one state of matter to another and these are provided below:
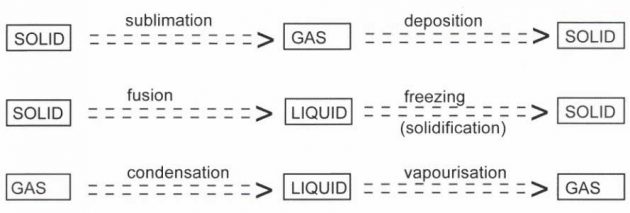
Energy is involved in the various changes of state that occur primarily in the form of heat. Each of the changes of state processes either uses heat or releases heat.
When a solid substance changes from the solid phase to the liquid phase, energy must be supplied in order to overcome the molecular attractions between the constituent particles of the solid. This energy must be supplied externally, normally as heat, and does not bring about a change in temperature. We call this energy latent heat (the word “latent” means “invisible”).
Two latent heats are typically described as follows. One is the latent heat of fusion (melting), and the other is the latent heat of vapourisation (evaporation).
Heat of fusion is the amount of thermal energy that must be absorbed or lost for 1 gram of a substance to change state from a solid to a liquid or vice versa. It is also called the latent heat of fusion (or the enthalpy of fusion) and the temperature at which it occurs is called the melting point.
When you withdraw thermal energy from a liquid or solid, the temperature falls. When you add heat energy the temperature rises. At the transition point between solid and liquid (the melting point), extra energy is required (the heat of fusion). To go from liquid to solid, the molecules of a substance must become more ordered. For them to maintain the order of a solid, extra heat must be withdrawn. ln the other direction, to create the disorder from the solid crystal to liquid, extra heat must be added.
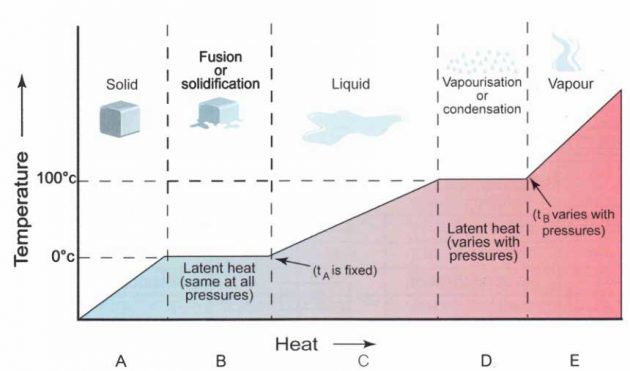
A: Rise in temperature as ice absorbs heat
B: Absorption of latent heat of fusion
C: Rise in temperature as liquid water absorbs
heat.
D: Water boils and absorbs latent heat of vaporisation.
E: Steam absorbs heat and thus increases its temperature
The heat of fusion can be observed if you measure the temperature of water as it freezes. lf you plunge a closed container of room temperature water into a very cold environment (say -20 °C), you will see the temperature fall steadily until it drops just below the freezing point (0 °C). The temperature then rebounds and holds steady while the water crystallises. Once completely frozen, the temperature will fall steadily again.
A change of state from liquid to vapour at constant temperature also requires the input of energy, called the latent heat of vapourisation (or standard enthalpy change of vapourisation). This implies that while a liquid undergoes a change to the vapour state at the normal boiling point, the temperature of the liquid will not rise beyond the temperature of the boiling point.
The latent heat of evaporation is the energy required to overcome the molecular forces of attraction between the particles of a liquid, and bring them to the vapour state, where such attractions are minimal.
When a liquid evaporates it cools. This ls because it is the higher energy molecules that leave the surface of the liquid, leaving lower energy molecules behind in the body of the liquid.
Gases – Properties and Rules
It is not only by alteration of its temperature that the volume of a gas can be changed. Changing the pressure exerted on it can also have this effect.
This tells us that a gas has three quantities – volume, temperature and pressure, all of which we now know may change. Early investigations of the physical properties of gases showed that, in certain respects, all the different gases behave in exactly the same way.
Boyles Law: The relationship between volume and pressure at constant temperature.
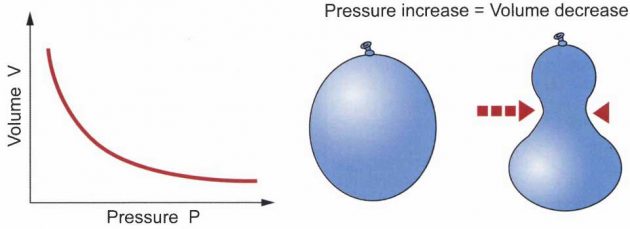
Boyle’s Law says that, for affixed amount of gas (fixed number of moles) at a fixed temperature, the pressure and the volume are inversely proportional.
pV = constant
“Moles is a unit of quantity in physical chemistry.”
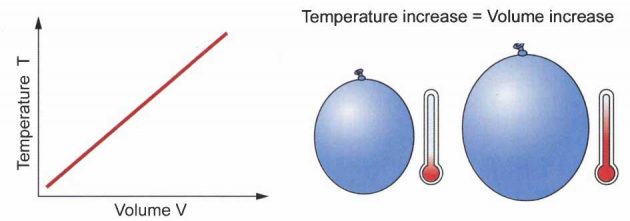
In other words, as the pressure increases, the volume decreases (when you squeeze on a balloon to increase the pressure, the volume of the balloon goes down).
Charles and Gay-Lussac’s Laws: the relationship between volume and temperature at constant pressure.
Charles Law says that for a fixed amount of gas (fixed number of moles) at a fixed pressure, the volume is proportional to the temperature.
VT = constant
In other words, as the temperature increases, the volume increases (when you heat a balloon the volume of the balloon goes up).
Gay-Lussac’s Law says that for a fixed amount of gas (fixed number of moles) at a fixed volume, the pressure is proportional to the temperature.
p/T = constant
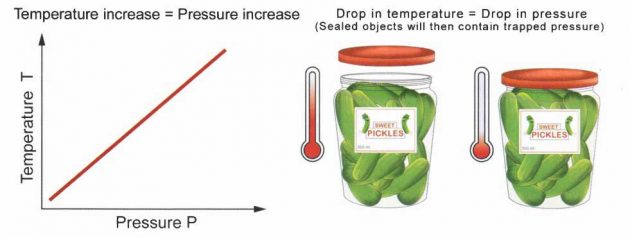
In other words, as the temperature increases, the pressure increases (when you put a pickle jar in the refrigerator, the drop in pressure from the trapped air becoming colder makes it hard to open the jar later!).
First Stage – Gas to Liquid
Gas can be moved using pipelines, but over long distances it becomes more economic to move it by ship. To ship gas, it must first be liquefied because it occupies a considerable amount of space at atmospheric pressure. As an example, the Basic Info about Liquefied Petroleum Gas Transportation on the LPG tankersliquefaction process shrinks natural gas to 600 times less than its original volume.
When a gas is compressed its molecules are forced closer together and, as their vibratory motion area is reduced, heat is given off. As compression proceeds, the speed of the molecules and the distances between them continues to decrease, until eventually the substance undergoes a change of state and becomes liquid.
Because of this effect, extreme cooling is not necessary during the liquefaction of gases. However, some level of cooling is required as each gas has a definite temperature, called its critical temperature, above which it cannot be liquefied regardless of the pressure exerted upon it.
A gas must be cooled to below its critical temperature before it can be liquefied.
As an example, to convert natural gas to a liquid it must be cooled to -82,5 °C to allow it to be compressed. However, the uneconomic and inefficient nature of this generally means that the main component, i. e. methane, is liquefied by refrigeration to -161,5 °C (-256 °F).
“These are the critical temperatures referred to for each gas in Table 1.”
The liquefaction process works much like a typical refrigerator. Cold liquid refrigerants such as propane and ethylene are reduced in pressure and evaporated as heat is exchanged with the natural gas steam. As this happens, it cools the natural gas to the point where it turns into a liquid. Once the gas has been liquefied, it is sent to storage to await shipping.
Gases and Vapours
As we are now dealing with a liquefied product, during shipping we must be concerned with the behaviour of the substance as a vapour rather than as a gas.
An important distinction between gases and vapours is that a gas is a substance in its gaseous state at a temperature above its critical temperature that cannot be liquefied by compression alone. However, a vapour is a substance in its gaseous state at a temperature that is below its critical temperature. For a vapour the critical pressure is the pressure required to compress a vapour to liquid at its critical temperature.
Saturated Vapour Pressure
The average energy of the particles in a liquid is governed by their temperature. The higher the temperature, the higher the average energy. But, within that average, some particles have energies higher than the average and others have energies that arc lower.
Some of the more energetic particles on the surface of the liquid can be moving fast enough to escape from the attractive forces holding the liquid together. This means they will evaporate.
The diagram shows a small region of a liquid near its surface.

Notice that evaporation only takes place on the surface of the liquid. This is quite different from boiling that happens when there is enough energy to disrupt the attractive forces throughout the liquid. It is the reason why, if you look at boiling water, you see bubbles of gas being formed all the way through the liquid.
If you look at water that is evaporating in the sun, you don’t see any bubbles. Water molecules arc simply breaking away from the surface layer. Eventually, the water will all evaporate in this way. The energy lost as the particles evaporate is replaced from the surroundings. As the molecules in the water jostle with each other new molecules will gain enough energy to escape from the surface.
The evaporation of a liquid in a closed container
Now imagine what happens if the liquid is in a closed container. Common sense tells you that water in a sealed bottle doesn’t seem to evaporate, or at least, it doesn’t disappear over time.
But there is constant evaporation from the surface. Particles continue to break away from the surface of the liquid, but this time they are trapped in the space above the liquid.

As the gaseous particles bounce around some of them will hit the surface of the liquid again and be trapped there. An equilibrium in which the number of particles leaving the surface is exactly balanced by the number re-joining it will rapidly be set up.

In this equilibrium there will be a fixed number of the gaseous particles in the space above the liquid.
When these particles hit the walls of the container, they exert a pressure. This pressure is called the saturated vapour pressure (also known as saturation vapour pressure) of the liquid.
A high vapour pressure means that the liquid must be volatile. Molecules escape from its surface relatively easily and aren’t very good at sticking back on again either resulting in larger numbers of them in the gas state once equilibrium is reached.
If you increase the temperature, you are increasing the average energy of the particles present. This means that more of them are likely to have enough energy to escape from the surface of the liquid, which will tend to increase the saturated vapour pressure.
Bubble Point
This is the temperature at which the first bubble of gas forms from a liquid. The bubble point temperature is usually lower than the dew point temperature.
Dew Point
Dew point is the condition where the system is all vapour, with only one (extremely small) drop of liquid present.
The condensing ratio gives an approximate indication of the quantity of liquid formed in dm3 (litre volume) when 1 m3 of vapour is compressed to a liquid under conditions of standard temperature and pressure, STP (at approximately 15 °C and 1 kg/cm2).
The critical temperatures, critical pressures and condensing ratios for the liquefied gases are listed in Table 1.
So, we can now logically see why, by varying the pressure above a liquid, it is possible to boil the liquid at different temperatures. Decreasing the pressure above the liquid lowers the boiling point. It is therefore possible, with this knowledge, to determine the saturated vapour pressure at various temperatures.
It can be seen from the graph at picture 14 that an increase in the temperature of the liquid causes a non-linear increase in the saturated vapour pressure.
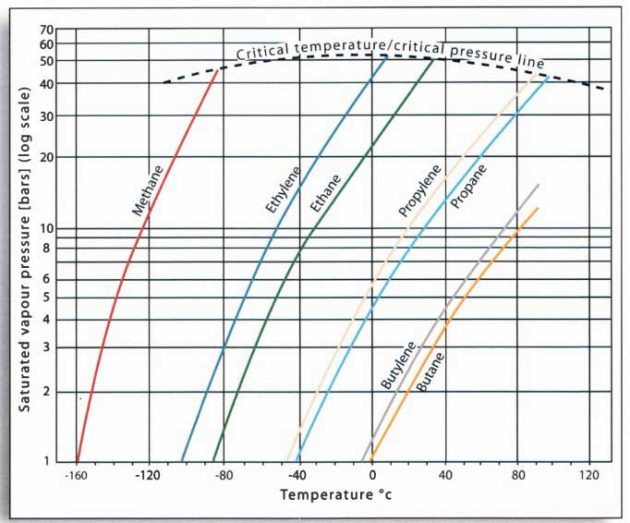
Different liquefied gases exert different vapour pressures, and you can now deduce from Table 1 that for the hydrocarbon gases, smaller molecules exert greater saturated vapour pressures than large ones and the unsaturated gases exert greater saturated vapour pressures than their equivalent saturated molecules.
You should also know that generally, the chemical gases exert lower saturated vapour pressures than the small hydrocarbon molecules.
The point of intersection of these curves with the horizontal axis indicates the boiling point of the liquid (the temperature at which the saturated vapour pressure is equal to an atmospheric pressure or 1 kg/cm2).
This is the temperature at which these cargoes would be transported in a fully refrigerated containment system.
“Whereas the kg/cm2 unit is frequently used for the measurement of pressure in the gas transport industry, a long standing recommendation has been to use kilo Pascals (kPa). Other units such as bar, millibar, pounds per square inch, or millimetres of either water or mercury may be encountered.”
Gauges are used for the measurement of pressure or to measure pressure difference. Gauge pressure is therefore the pressure difference between the pressure inside the apparatus and the pressure outside.
A liquefied gas may be defined in terms of its pressure as a substance whose vapour pressure at 37,8 °C, the Reid Vapour Pressure test, is equal to or greater than 2,8 kg/cm2 (although earlier in this article and in the international Gas Code, a value of 2,8 barAbs is referred to – a small difference in actual measurement).
Liquid and Vapour Densities
The density of a liquid (or its specific gravity) is defined as the mass per unit volume and is commonly measured in kilogramme per cubic decimeter (kg/dm3, or litre).
The variation of the density of a boiling liquid (propylene) with temperature is shown in picture 15 (curve yi) where yi gamma is the specific gravity of the boiling liquid and yii gamma is the specific gravity of the saturated vapour. The liquid is expressed in kilograms per decimetre (litres) and the vapour in kilograms per cubic metre.
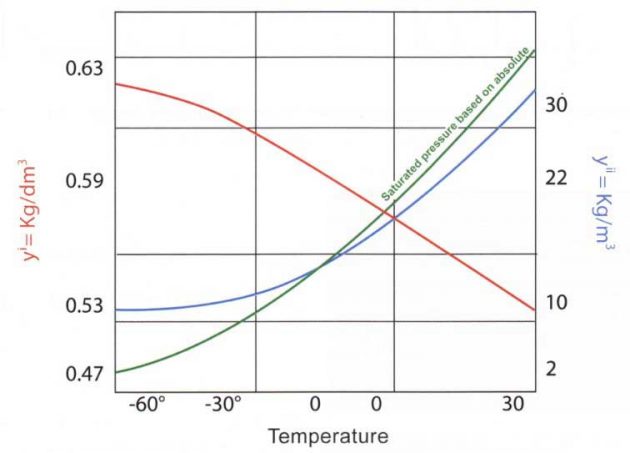
As can be seen, the density of a liquid decreases with increasing temperature. This means that, as the liquid is heated, its volume increases but the mass remains constant.
Values for the density of boiling liquefied gases relative to water (which is taken to be 1) at a standard temperature and pressure, were provided in Table 1.
“This means that in the event of a spillage onto water these liquids (other than chlorine) will float prior to evaporation.”
As can be seen, all liquefied gases with the exception of chlorine have liquid relative densities less than 1.
Density of the vapour is commonly quoted in units of kilogrammes per cubic metre (kg/m3).
The density of saturated vapour increases with increasing temperature. This is because the vapour is in contact with its originating liquid and, as the temperature rises, more liquid transfers into the vapour phase. This results in a considerable increase in mass, relative to the increase in volume.
The densities of various vapours relative to air (which is taken to have a value of 1), at standard temperature and pressure, are shown in Table 1. As can be seer, all the liquefied gases produce vapours that have a relative vapour density greater than 1, with the exception of methane (at temperatures greater than -100 °C) and ammonia.
“This means that most vapours from spillages tend to seek lower ground and do not disperse readily with the exception of ammonia and/or methane vapours, which will seek lower-ground when cold and then heat up and rise to higher levels.”
The liquid and vapour densities can be used in cargo calculations to determine the mass of cargo in a particular tank at a specified temperature.
Effect of Pressure on Containment
Knowing the pressure that a liquid or gas exerts at a temperature of 37,8 °C provides important information about how a cargo should be contained.
For example, propane exerts approximately 13,5 bar Absolute at 37,8 °C and has either to be contained in full pressure containment piping and tanks, refrigerated to a colder temperature around its boiling point of -42 °C or contained within part pressure and some part refrigeration. Similarly, normal butane exerts approximately 3,5 bar Abs at 37,8 °C, and could be refrigerated or cooled to -1 °C to bring it down to its boiling point.
These absolute pressures can be related to atmospheric by reducing approximately 1 bar, so the gauge pressures of the examples shown above would be about 12,5 barg for propane and 2,5 barg for the n-butane.
Refrigeration
A highly volatile liquid, such as a liquefied gas, when first passed along a pipe or into a tank vessel can cool to a temperature that is well below the temperature of the liquid.
As an example (see picture 16), LPG vapour from a tank is compressed to a liquid and passes across an expansion valve after a cold condensing unit. It vaporises on passing through the expansion valve to the tank, which is at low pressure. On evaporator, the liquid takes heat from the surroundings.
The vapour that is compressed readily exchanges its heat in the liquid cooled condenser. lf the system was 100 % efficient, then the heat gained from the compressor would be equal to the heat lost downstream from the expansion valve.
Lubrication and Viscosity
Viscosity is commonly perceived as “thickness” or resistance to pouring. It describes a fluid’s internal resistance and may be thought of as a measure of fluid friction. The viscosity of a liquid is important in determining its lubricating properties.
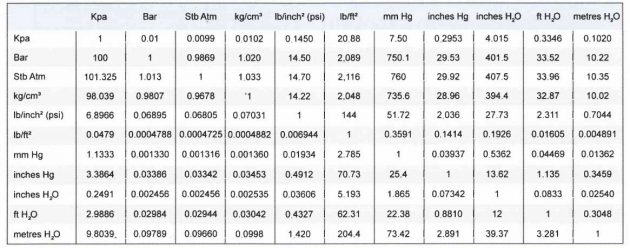
“While liquefied gases offer poor lubricating properties for pumping, they do offer excellent cooling properties.”
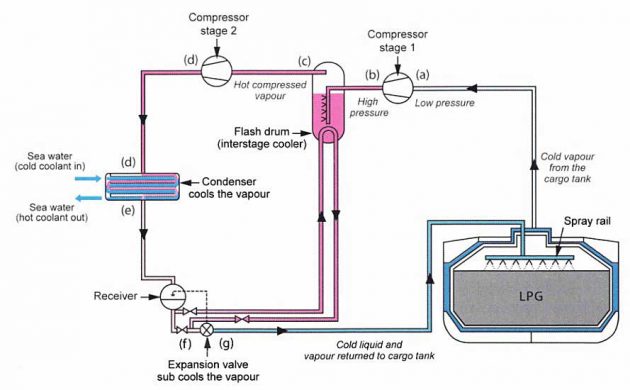
The majority of liquefied gases have poor lubricating properties when compared with lubricating oils, or even water. This is an important point when operating deep well pumps as the drift bearings are lubricated by the cargo liquid and any heating of these bearings, especially when stripping a tank, could cause both damage and gassing of the liquid.
Temperature Conversion
Although the vast majority of literature and regulatory information on the carriage of liquefied gases follows the agreed use of the Celsius scale of temperature, there are operators, mainly in the USA, who continue to use the Fahrenheit scale. A temperature conversion table is shown as Table 3.

What are the Gases Carried on Liquefied Gas Carriers Used For?
The ship has no involvement with the gas after it has been discharged, but to help in your knowledge of the cargoes carried on these ships, the following overview provides an indication of what the gases carried are commonly used for:
1 LNG (Methane/Ethane) (CH4/C2H6)
Methane is important for electrical generation, burning it as a fuel in gas turbines or steam boilers. When compared to other hydrocarbon fuels, burning methane produces less carbon dioxide for each unit of heat released. Also, methane’s heat of combustion is lower than any other hydrocarbon. Methane in mixture with air releases a very clean flame with fewer particles than other hydrocarbons.
Methane is piped into homes for domestic heating and cooking purposes. In the USA more than 60 million Americans use it to heat their homes.
2 Propane (C3H8)
6,5 million households use propane as their primary heating fuel in the USA alone. Propane also powers motor vehicles, buses, forklifts, and taxis and it is increasingly used as a vehicle fuel. Propane used in vehicles is known as “Autogas” and about 9 million vehicles worldwide use it.
“CAUTION: Propane used in America is different to the “propane” sold overseas, which is actually a mixture of propane and butane. The warmer the country, the higher the butane content, commonly 50/50 and sometimes reaching 75 % butane.”
Another use of propane is as a propellant for aerosol sprays, especially now there is a ban on the use of CFCs.
Propane is the fastest growing fuel source in countries such as China and India.
3 Butane (C4H10)
Normal butane gas is sold bottled as a fuel for cooking and camping, where it is referred to commercially as LPG. It is also used as a petrol component, as a feedstock for the production of base petrochemicals in steam cracking, as a fuel for cigarette lighters and is also used as a propellant in aerosol sprays. Iso-butane is a valuable feedstock in chemical and gasoline additives.
4 Ethylene (C2H4)
Ethylene is used primarily as an intermediate in the manufacture of other chemicals, especially plastics. Ethylene may be polymerised directly to produce polyethylene (also called polyethene or polythene), the world’s most widely-used plastic.
Ethylene can be chlorinated to produce ethylene dichloride (1,2-Dichloroethane), a precursor to the plastic polyvinyl chloride, or combined with benzene to produce ethylbenzene, which is used in the manufacture of polystyrene, another important plastic.
“Ethylene usage is a barometer of the world economy because of its key role in the production of plastic goods.”
Smaller amounts of ethylene are oxidized to produce chemicals including ethylene oxide, ethanol, and polyvinyl acetate.
Global demand for ethylene exceeded 100 million metric tonnes per year in 2005. Ethylene is also used as a “ripening” agent in the chilled fruits market.
5 Propylene (C3H6)
The main use of propylene is in the production of polypropylene.
6 Butylene (C4H8)
Used in the production of synthetic rubber in addition to other uses with petrochemical intermediates. Isobutylene is also used for the production of isooctane, which improves the combustion qualities of gasoline.
7 Butadiene (C4H6)
Most butadiene is polymerised to produce synthetic rubber. While polybutadiene itself is a very soft, almost liquid material, polymers prepared from mixtures of butadiene with styrene or acrylonitrile, such as ABS, are both tough and elastic. Styrene- butadiene rubber is the material most commonly used for the production of automobile tyres.
Smaller amounts of butadiene are used to make nylon and other synthetic rubber materials such as chloroprene.
8 Vinyl Chloride Monomer/VCM
Vinyl chloride is manufactured on an industrial scale, from ethylene and chlorine in the presence of iron chloride as a catalyst. VCM’s prime use is to make PVC.
9 Anhydrous Ammonia (NH3)
The most important single use of ammonia is in the production of nitric acid for use in the production of fertilizers and explosives. In addition to serving as a fertilizer ingredient, ammonia can also be used directly as a fertilizer by forming a solution with irrigation water, without additional chemical processing. This allows the continuous growing of nitrogen dependent crops such as maize (corn) without crop rotation, although it leads to poor soil health.
Ammonia has thermodynamic properties that make it very well suited as a refrigerant. It liquefies readily under pressure so it was used in virtually all refrigeration units prior to the advent of halo-alkanes, such as freon.
However, ammonia is a toxic irritant and its corrosiveness to any copper alloys increases the risk that an undesirable leak may develop and cause a noxious hazard. Its use in small refrigeration units has been largely replaced by halo-alkanes, which are not toxic irritants and are practically not flammable. Ammonia continues to be used as a refrigerant in large industrial processes such as bulk ice making and industrial food processing.
10 Chlorine (Cl)
Chlorine is an important chemical for some processes of water purification, in disinfectants and in bleaches.
Chlorine is widely used in the manufacture of many every-day items, or to purify water in various forms. It is used (in the form of hypochlorous acid) to kill bacteria and other microbes from drinking water supplies and swimming pools. Even small water supplies are now routinely chlorinated.
Chlorine is also used widely in paper product production, antiseptic, dyestuffs, food, insecticides, paints, petroleum products, plastics, medicines, textiles, solvents, and many other consumer products.