Uncover the transformative innovations and diverse applications of M2M technology in this comprehensive article. Explore how M2M is revolutionizing industries through seamless connectivity, real-time data exchange, and enhanced operational efficiency.
- A General Overview of the Internet of Things and M2M
- M2M Frameworks
- M2M Applications Examples and Satellite Support
- Examples of General Applications
- Satellite Roles, Context, and Applications
- Antennas for Satellite M2M Applications
- M2M Market Opportunities for Satellite Operators
- Key Satellite Industry Players and Approaches
- Competitive Wireless Technologies
- Universal Mobile Telecommunications System (UMTS)
- Long-Term Evolution (LTE)
In this article, we first provide a generic overview of the emerging Internet of Things (IoT) and a contemporary perspective on machine-to-machine (M2M) communications, then we focus on satellite support of these important requirements for those environments where satellites can provide an optimal solution. According to industry watchers, the IoT has the potential to sustain an added global economic value of $6 trillion annually by 2025; satellite’s share is currently seen in being “a few billion dollars,” however, the opportunity for a larger role for satellite-based services exists. With M2M technology that enables automated data exchanges between devices, organizations can track, monitor, and manage global assets; just-in-time inventories; enterprise fleets; energy grids; pipelines; mining operations; remote infrastructure; emergency operations; personnel deployments; and natural processes such as weather, ecology, farming, forestry, and water flows. The intrinsic satellite-based M2M value proposition is that “global businesses need access to global data.” Satellite operators, for example, Iridium, make the point that global pole-to-pole connectivity services can extend M2M to the 90 % of the earth not serviced by terrestrial networks.
A General Overview of the Internet of Things and M2M
The Some portion of this material are summarized from reference.x Services, service options, and service providers change over time (new ones are added and existing ones may drop out as time goes by); as such, any service, service option, or service provider referred to in this article is mentioned strictly as illustrative examples of possible examples of emerging technologies, trends, or approaches. As such, the mention is intended to provide pedagogical value. No recommendations are implicitly or explicitly implied by the mention of any vendor or any product (or lack thereof).x proliferation of an ever-growing set of devices that are able to be directly connected to a data network or to the Internet is leading to a new ubiquitous-computing paradigm: the opportunity now exists to connect all “things” and objects that have (or will soon have) embedded wireless (or wireline) connectivity to control systems that support data collection, data analysis, decision-making, and (remote) actuation.
In the IoT, commonly deployed devices and objects contain an embedded device or microprocessor that can be accessed by some communications mechanism, typically utilizing wireless links. “Things” include, but are not limited to, machinery, home appliances, vehicles, individual persons, pets, cattle, animals, habitats, habitat occupants, as well as enterprises.
Interactions are achieved utilizing:
- A plethora of possibly different networks;
- Computerized devices of various functions, form factors, sizes, and capabilities – such as iPads, smartphones, monitoring nodes, sensors, and tags;
- A gamut of host application servers.
This new paradigm seeks to enhance the traditional Internet (or intranet) into an ecosystem created around intelligent interconnections of diverse objects in the physical world. The IoT aims at closing the gap between objects in the material world, the “things,” and their logical representation in information systems. The “things” are also variously known as “objects,” “devices,” “end nodes,” “remotes,” or “remote sensors,” to list just a few commonly used terms.
The IoT generally utilizes low-cost information gathering and dissemination devices – such as sensors and tags – that facilitate fast-paced interactions in any place and at any time, among the objects themselves, as well as among objects and people; actuators are also part of the IoT. Hence, the IoT can be described as a new-generation information network that enables seamless and continuous M2M Some (e. g., 3GPP) also use the term Machine-Type Communications (MTC) to describe M2M systems.x and/or human-to-machine (H2M) communication.
One of the initial goals of the IoT is to enable connectivity for the various “things,” allowing some form of interrogation of the “thing’s” status or some local variable; a next goal is to be able to have the “thing” provide back appropriate, application-specific telemetry, perhaps based on Artificial Intelligence (AI) principles; an intermediary next step is to provide a web-based interface to the “thing” (especially when human access is needed); the final step is to permit actuation by the “thing” (i. e., to cause a function or functions to take place either under external control or with intrinsic AI mechanisms). Certain “things” are stationary, such as an appliance in a home; other “things” may be in motion, such as a car, a container, a carton (or even an item within the carton) in a supply chain environment (either end-to-end or while in an intermediary warehouse).
Thus, the two fundamental components of the IoT are:
- Intelligence (microprocessing) to be embedded into the various “things,” and;
- A network or a collection of networks (the first hop of which is almost invariably wireless) to connect the “things” to the rest of the world.
In general, “things” have the following characteristics, among others:
- Have the ability to sense and/or actuate (but not always);
- Are generally small (but not always);
- Have limited computing capabilities (but not always);
- Are energy/power limited (but not always);
- Are related to the physical world;
- Are mobile (but not always);
- Sometimes have intermittent connectivity;
- Managed by devices, not people (but not always);
- Of interest to people.
For the purpose of this specific satellite-oriented discussion, objects (“things”) tend to be cargo or an airplane/ship engine systems/subsystems; however, other “things” are not excluded.
As a point of observation, the Institute of Electrical and Electronics Engineers (IEEE) Computer Society stated that:
The Internet of Things (IoT) promises to be the most disruptive technology since the advent of the World Wide Web. Projections indicate that up to 100 billion uniquely identifiable objects will be connected to the Internet by 2020, but human understanding of the underlying technologies has not kept pace. This creates a fundamental challenge to researchers, with enormous technical, socioeconomic, political, and even spiritual, consequences. IoT is just one of the most significant emerging trends in technology.
The European Commission recently made these observations, which we can employ in our discussion of the IoT:
Considering the functionality and identity as central it is reasonable to define the IoT as “Things having identities and virtual personalities operating in smart spaces using intelligent interfaces to connect and communicate within social, environmental, and user contexts”. A different definition, that puts the focus on the seamless integration, could be formulated as “Interconnected objects having an active role in what might be called the Future Internet”. The semantic origin of the expression is composed by two words and concepts: “Internet” and “Thing,” where “Internet” can be defined as “The world-wide network of interconnected computer networks, based on a standard communication protocol, the Internet suite (TCP/IP),” while “Thing” is “an object not precisely identifiable” Therefore, semantically, “Internet of Things” means “a world-wide network of interconnected objects uniquely addressable, based on standard communication protocols”.
Figure 1 depicts the high-level logical partitioning of the interaction space; this figure illustrates human-to-human (H2H) communication, M2M communication, H2M communications, and machine in (or on) humans (MiH) communications [MiH devices may include chips embedded in humans, medical monitoring probes, and Global Positioning System (GPS) bracelets].
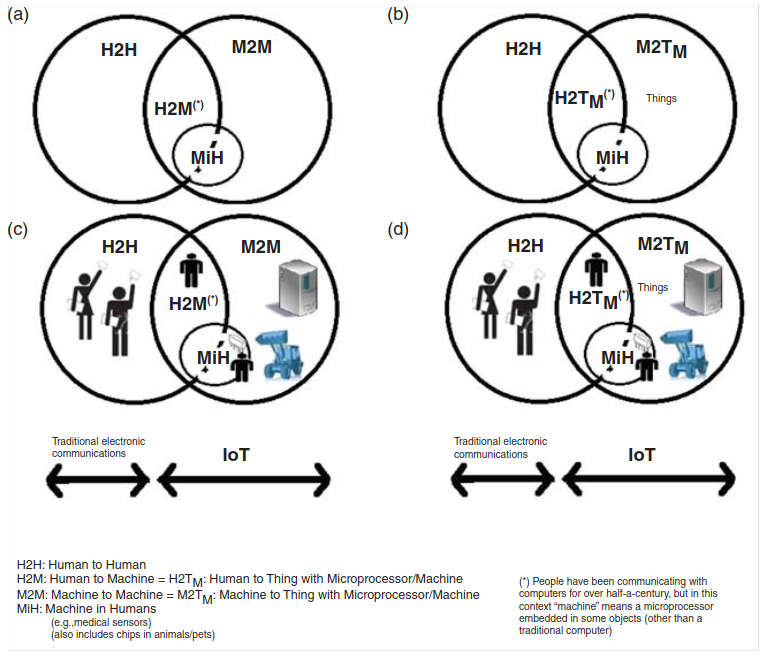
(a) Interaction space partitioning showing humans and machines. (b) The target machine is shown explicitly to be embedded in the “thing.” (c) Interaction space showing icons. (d) Embedded machine, icon view
Recently, the IoT has been seen as an emerging “paradigm of building smart communities” through networking of various devices enabled by M2M technologies (but not excluding H2M), for which standards are now emerging. As a general concept, the IoT effectively eliminates time and space isolation between geographical space and virtual space, forming what proponents label as “smart geographical space” and creating new human-to-environment (and/or H2M) relationships. The latter implies that the IoT can advance the goal of integration of human beings with their surroundings. A smart environment can be defined as consisting of networks of federated sensors and actuators, and can be designed to encompass homes, offices, buildings, and civil infrastructure; from this granular foundation, large-scale end-to-end services supporting smart cities, smart transportation, and Smart Grids (SGs), among others, can be contemplated.
The IoT is a general, heterogeneous concept with somewhat open-ended definitions; M2M, on the other hand, is a tighter well-defined concept (a subset of the IoT), where standards have evolved and have been published by industry organizations such as European Telecommunications Standards Institute (ETSI). The focus of the IoT is on M2M, H2M, and MiH applications (also see Table 1).
Table 1. Taxonomy of “Things” in IoT | ||||||||||
---|---|---|---|---|---|---|---|---|---|---|
H2M (Human to Machine) | ||||||||||
Data integration point or person (DIPP) “thing” | H | Stationary access/connectivity | Local mobility access/connectivity | Full mobility access/connectivity | ||||||
“Remote thing” also known as data end point (DEP) | M | Target device is stationary | Target device has local mobility | Target device has full mobility | Target device is stationary | Target device has local mobility | Target device has full mobility | Target device is stationary | Target device has local mobility | Target device has full mobility |
Example | Access a home thermostat from an office PC | Access a monitor on a home-bound pet from an office PC | Access a home thermostat from a home, office, or hotspot wireless PC | Access a home thermostat from a home, office, or hotspot wireless PC | Access a monitor on a home-bound pet from a home, office, or hotspot wireless PC | Access a GPS device on a teenager’s car from a home, office, or hotspot wireless PC | Access a home thermostat from a smartphone | Access a monitor on a homebound pet from a smartphone | Access a GPS device on a teenager’s car from a smartphone | |
M2M (Machine to Machine) | ||||||||||
DIPP “thing” | M1 | Stationary access/connectivity | Local mobility access/connectivity | Full mobility access/connectivity | ||||||
Remote “thing“ | M2 | Target device is stationary | Target device has local mobility | Target device has full mobility | Target device is stationary | Target device has local mobility | Target device has full mobility | Target device is stationary | Target device has local mobility | Target device has full mobility |
Example | Access a home electrical meter from an office/provider server | Access a monitor on a home-bound pet from an office/provider server | Access a GPS device on a person’s car from an office/provider server Network Operation Center access to an airplane engine via satellite | Access a home electrical meter from a WLAN-based office/provider server | Access a monitor on a home-bound pet from a WLAN-based office/provider server | Access a GPS device on a person’s car from a WLAN-based office/provider server | Access a home electrical meter from roaming-3G/4G based provider server | Access a monitor on a home-bound pet from a roaming-3G/4G based provider server | Access a GPS device on a person’s car from a roaming-3G/4G based provider server Military in-theater access to an UAV from a vehicle via satellite |
M2M services aim at automating decision and communication processes and support consistent, cost-effective interaction for ubiquitous applications (e. g., fleet management, smart metering, home automation, e-health). M2M communications per se is the communication between two or more entities that do not necessarily need direct human intervention: it is communication between remotely deployed devices with specific roles and requiring little or no human intervention. M2M communication modules are usually integrated directly into target devices, such as Automated Meter Readers (AMRs), vending machines, alarm systems, surveillance cameras, and automotive/aeronautical/maritime equipment, to list a few. These devices span an array of domains including (among others) industrial, trucking/transportation, financial, retail Point of Sales (POS), energy/utilities, smart appliances, and healthcare. The emerging standards allow both wireless and wired systems to communicate with other devices of similar capabilities; M2M devices, however, are typically connected to an application server via a mobile data communications networks.
At the “low end” of the spectrum, the thing’s information is typically stored in a Radio Frequency Identification (RFID) electronic tag; and the information is uploaded by noncontact reading using an RFID reader. At the “mid range” of the spectrum, one finds devices with embedded intelligence (microprocessors) and embedded active wireless capabilities to perform a variety of data gathering and, possibly, control functions. Automatic on-body biomedical measurements, home appliance and power management, and industrial control are some examples of these applications.
At the other end of the spectrum, more sophisticated sensors can also be employed in the IoT: some of these sensor approaches use distributed Wireless Sensor Networks (WSNs) systems that:
- Can collect a wide variety of environmental data such as temperature, atmospheric and environmental chemical content, engine status, homeland security applications [e. g., geofencing, unmanned aerial vehicles (UAV)], or even low- or high-resolution ambient video images from geographically dispersed locations;
- Can optionally preprocess some or all of the data, and;
- Can forward all this information to a centralized (or distributed/virtualized) site for advanced processing.
These objects may span a city, region, or large distribution grid; or they may be mobile devices associated with airplane or ship engines or process control systems on oil well (on land or in the water).
Eight specific vertical market segments recognized at press time included the following: utilities and SG; automotive and transportation; logistics; public safety; security and surveillance; retail and vending; healthcare; intelligent buildings and smart cities; and consumer electronics.
More specifically, the following applications are of interest in the satellite context:
- Remote structures, such as water-deployed Oil & Gas (O&G) platforms, O&G pipelines, power transmission lines, sparse geofencing structures (e. g., sensors on national borders), and mining, forestry, and agricultural sensors. These structures include in-water oil wells throughout the world, land-based pipelines traversing long rural and sparsely inhabited regions, and dispersed power lines. Energy management functions fit under this category, as well as environmental protection activities, and homeland security endeavors. Often it is impossible to have cellular or other wireless coverage in many exurban or mountainous environments and satellite solutions are ideal.
- Land mobile platforms, including vehicles or structures at a fixed location or in motion. Vehicles can be automobiles, trucks, and military equipment. While cellular coverage may be available, vehicles traveling a long distance may find that a homogeneous solution that works end-to-end is preferable. Fleet management functions fit under this category.
- Maritime platforms, including vessels traveling on a body of water, especially in oceans. These vessels may include merchant ships, tankers, container ships, fishing vessels, cruise ships, ferries, pleasure yachts (>60 ft) and superyachts (>120 ft), Coast Guard ships, and buoys.
- Aeronautical platforms, including commercial and business airplanes, helicopters, UAVs, military planes (the military may have its own systems, but they also rely on commercially provided services).
- Civil infrastructure management (cities, traffic, resources) in Third World countries (and/or rural geographies) where modern connectivity systems may still be rudimentary.
Figures 2 and 3 provide pictorial views of typical IoT applications; these figures only depict illustrative cases and are not exhaustive or normative.
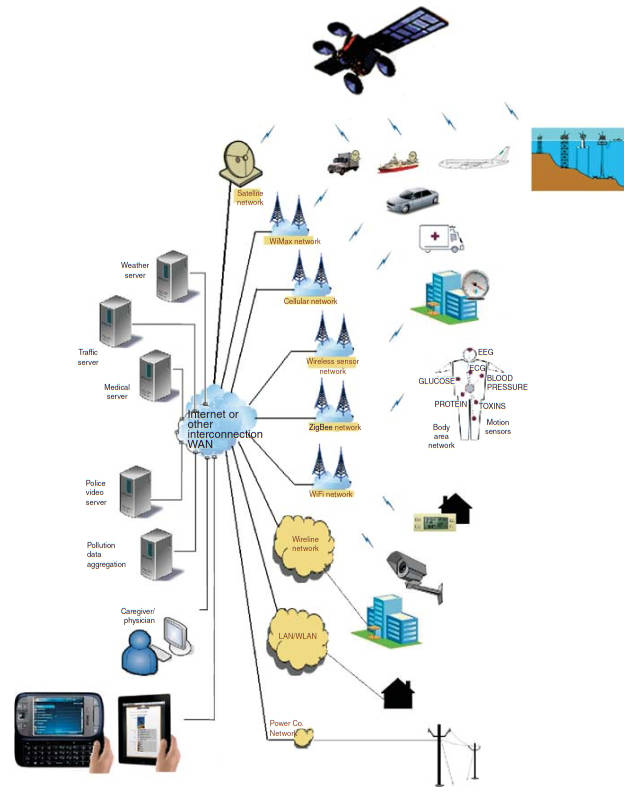
Note that personal communication devices (smartphones, pads, etc.) can be viewed as machines or just simply as end nodes; when personal communication devices are used for H2M devices where the human employs the smartphone to communicate with a machine (such as a thermostat or a home appliance), then we consider the personal communication devices part of the IoT (otherwise we do not).
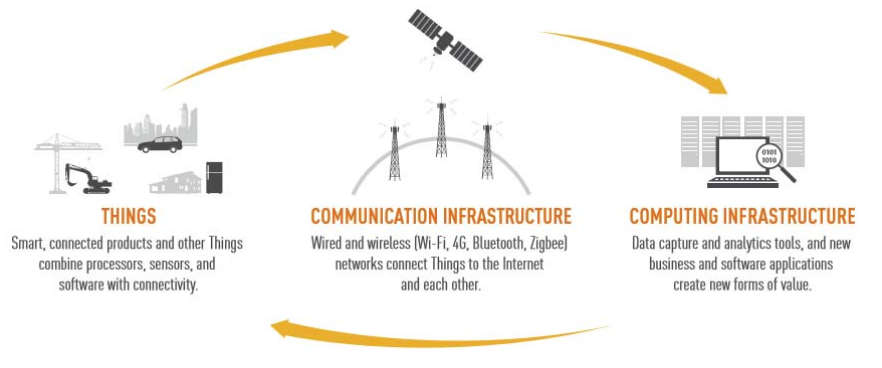
It has been estimated that in 2011 there were 7 billion people on earth and 60 billion machines worldwide. Market research firm Frost & Sullivan recently forecasted that total cellular M2M connections are expected to increase from around 24 million in 2010 to more than 75 million by 2017; worldwide, the expectation is that the number of M2M device connections will grow from around 60 million in 2010 to over 2 billion in 2020.
Satellite-based M2M systems are currently in the range of 1–2 million by the number of deployed units. Other market research puts the worldwide M2M revenues at over $38 billion in 2012. Yet other market research companies project 15 billion connected devices moving 35 trillion gigabytes of data at a cost of $3 trillion annually by 2015. Other market research sees the wireless M2M market as accounting for nearly $196 billion in annual revenue by the end of 2020, following a Compound Annual Growth Rate (CAGR) of 21 % during the 6-year period between 2014 and 2020; these market estimates also see the installed base of M2M connections (wireless and wireline) as growing at a CAGR of 25 % between 2014 and 2020, eventually accounting for nearly 9 billion connections worldwide. These market data point to major development and deployment of the IoT technology in the next few years.
It should be noted that the characteristics of IoT/M2M communication are often different from other types of networks or applications. For example, cellular mobile networks are designed for human communication and communication is connection centric; this kind of communication entails interactive communication between humans (voice, video), or data communication involving humans (web browsing, file downloads, etc.); it follows that cellular mobile networks are optimized for traffic characteristics of human-based communication and applications. On the other hand, in M2M environment the expectation is that there are many devices, there will be long idle intervals, transmission typically entails small messages, there may be relaxed delay requirements, the data are coded in defined codepoints or are raw measurements, and the device’s energy efficiency is, generally, paramount.
Table 2 depicts some key properties and requirements of M2M applications.
Table 2. Properties and Requirements of M2M Applications | ||||||
---|---|---|---|---|---|---|
Land Mobile | Aeronautical | Maritime | eHealth | Surveillance | Smart Meters | |
Mobility | Vehicular medium/long distance | High-speed long distance | Low-speed long distance | Pedestrian/vehicular | None | |
Message size | Small – Medium | Medium | Large | Small (few kB) | ||
Traffic pattern | Periodic/Irregular | Periodic | ||||
Device density | Medium | Low | Very high | |||
Latency requirements | Medium(s) | Medium (<200 ms) | Low (up to hours) | |||
Power efficiency requirements | Medium | High (battery power devices) | Low | High (battery powered meters) | ||
Reliability | High | Medium | High | |||
Security requirements | High | Very high | Medium | High |
A number of wireless technologies to support M2M are available, which are as follows:
- Personal Area Networks (PANs): Zigbee®, Bluetooth®, especially Bluetooth Low Energy (BLE), Near Field Communications (NFCs), and proprietary systems; specifically, there is interest in Low-power Wireless Personal Area Networks (LoWPANs); some of these PANs are also classified as Low-Rate Wireless Personal Area Networks (LR-WPANs);
- Wireless Local Area Networks (WLANs): Wi-Fi® IEEE Standard 802.11 (including vendor-specific implementations for low power In recent years, several improvements have been made to the Wi-Fi LAN standard; some of these improvements (including IEEE Standard 802.11v) are aimed at reducing its power consumption. Wi-Fi is optimized for traditional Office Automation (OA) of large data transfer where high throughput is needed; it is not generally intended for coin cell operation.x);
- Metropolitan Area Networks (MANs): WiMAX;
- WSN: application-specific technology, in general;
- 3G/4G cellular: Universal Mobile Telecommunications System (UMTS), General Packet Radio Service (GPRS), Enhanced Data rates for GSM Evolution (EDGE), Code Division Multiple Access (CDMA-2000/EV-DO), Wideband CDMA (W-CDMA/HSPA), and Long-Term Evolution (LTE);
- Satellite (GEO, MEO, LEO) networks for remote, widespread, hostile, or global environments, and;
- Hybrid (e. g., satellite backhaul of cellular systems) for developing environments.
While IoT/M2M connectivity might be achieved by wireline means, for example, PLC (Power Line Communication)-based grid management, some operators have used wireless technology for meter reading. Furthermore, although energy suppliers routinely utilize Supervisory Control and Data Acquisition (SCADA)-based systems to enable remote telemetry functions in the power grid, and although, traditionally, SCADA systems have used wireline networks to link remote power grid elements with a central operations center, at this time an increasing number of utilities are turning to public cellular networks to support these functions.
Due to its global reach and the ability to support mobility in all geographic environments (including Antarctica), satellite communications can play a critical role in many broadly distributed M2M applications. While some background on other wireless technologies is provided at the end of the article, the core of this discussion is on satellite communication. In the satellite arena, M2M has generally been supported by Mobile Satellite Service (MSS) approaches. As we have seen in previous chapters, MSS deals with the delivery of services (data streams) to portable and mobile terminals with a variety of (small) antennas. These terminals are typically found on O&G, land mobile, maritime, and aeronautical platforms. Satellite M2M players include Iridium, Inmarsat, Orbcomm, and Globalstar. M2M is considered to be a value-added service and equipment market, rather than a simple capacity-only business, which some satellite operators seem to thrive on.
As noted, various technologies have indeed emerged in the past two decades that can be utilized for implementations including PANs, such as IEEE 802.15.4; WLANs; WSNs; 3G/4G cellular networks; satellite networks; metro-Ethernet networks; MultiProtocol Label Switching (MPLS); and Virtual Private Network (VPN) systems. Wireless access and/or wireless ad hoc mesh systems reduce the “last mile” cost of IoT applications for distributed monitoring and control applications. Satellite communication also can play a significant role for the five classes of application listed earlier (remote structures, land mobile, maritime, aeronautical, civil infrastructure in the Third World), especially for unconnected/undeveloped/underdeveloped environments. On a different vein, we believe that the fundamental technical advancement that will foster the deployment of the IoT is Internet Protocol version 6 (IPv6). In fact, IoT may well become the “killer-app” for IPv6. IoT is deployable using IP version 4 (IPv4) as has been the case in the recent past, but only IPv6 provides the proper scalability and functionality to make it economical, ubiquitous, and pervasive. There are many advantages in using IP for IoT, but we have to ascertain that the infrastructure and the supporting technology scale to meet the challenges. This is why there is broad agreement that IPv6 is critical for the deployment of the IoT.
The reader should realize, however, that despite significant technological advances in many subtending disciplines, difficulties associated with the evaluation of IoT solutions under realistic conditions in real-world experimental deployments still hamper their maturation and significant rollout. Obviously, with limited standardization, there are capability mismatches between different devices; also there are mismatches between communication and processing bandwidth. Although IoT systems can utilize existing Internet protocols, in a number of cases the power-, processing, and capabilities-constrained IoT environments can benefit from additional protocols that help optimize the communications and lower the computational requirements. The M2M environment has been a fragmented space, but recent standardization efforts are beginning to show results. Standards covering many of the underlying technologies are critical because proprietary solutions fragment the industry. Standards are particularly important when there is a requirement to physically or logically connect entities across an interface. Device, network, and application standards can enable global solutions for seamless operations at reduced costs. Specifically proponents make the case that IPv6 is the fundamental optimal network communication technology to deploy IoT in a robust, commercial manner rather than just a preliminary desktop “science experiment” in some academic researcher’s lab (layer 2 wireless technologies are also critical to IoT’s end-to-end connectivity).
IoT standardization spans several domains including physical interfaces, access connectivity (e. g., low-power IEEE 802.15.4-based wireless standards such as IEC62591, 6LoWPAN, and ZigBee Smart Energy (SE) 2.0, DASH7, ETSI M2M), networking (such as IPv6), and applications. Some studies have shown that for the home two wireless physical layer communications technologies that best meet the overall performance and cost requirements are Wi-Fi (802.11/n) and ZigBee (802.15.4). Examples of standardization efforts targeted for these environments include the initiatives known as “Constrained RESTful Environments (CoRE),” “IPv6 over Low power WPAN (6LoWPAN),” and “Routing Over Low power and Lossy (ROLL) networks,” which have (and are being) studied by appropriate Working Groups of the IETF.
M2M Frameworks
This section elaborates on the concept, definition, and a usable framework of the IoT. We noted above that the IoT is an evolving type of Internet application that endeavors to make a thing’s information (whatever that may be) securely available on a global scale if/when such information is needed by an aggregation point or points. M2M systems have enjoyed some (useful) standardization in recent years.
Some applicable observations related to M2M include the following:
The International Telecommunications Union, Telecommunications (ITU-T) is in the process of identifying a common way to define/describe the IoT. So far, the ITU-T has not found “a good definition to cover all aspects of IoT as the IoT has quite big scope not only the technological viewpoints but also other views … We recognized whatever we define, everyone cannot be happy”. One can view the Internet as an infrastructure providing a number of technological capabilities or as a concept providing an array of data exchange and linkage services. The infrastructure perspective describes the Internet as a global system of interconnected computer networks (of many conceivable technologies) that use the TCP/IP suite to communicate; the networks comprise millions of private, public, business, academic, and governmental servers, computers, and nodes. One can view the Internet as a concept. The concept perspective sees the Internet as a worldwide logical interconnection of computers and networks that support the exchange of information among users, including but not limited to interlinked hypertext documents of the World Wide Web (WWW).
While the IoT can, in principle, be seen as a more encompassing concept than what is captured under the ETSI M2M standards and definitions, nonetheless, the M2M definitions can serve the purpose of adding some structure to the discussion of the general IoT environment.
From Figure 1 it has been noted that a high-level logical partitioning of the entity-to-entity interaction space included H2H communication, M2M communication, H2M communications, and machine in (or on) humans (MiH) communications (MiH devices may include medical monitoring probes, and GPS bracelets). Figure 4 illustrates classes of generic IoT arrangements that are included in our discussion.
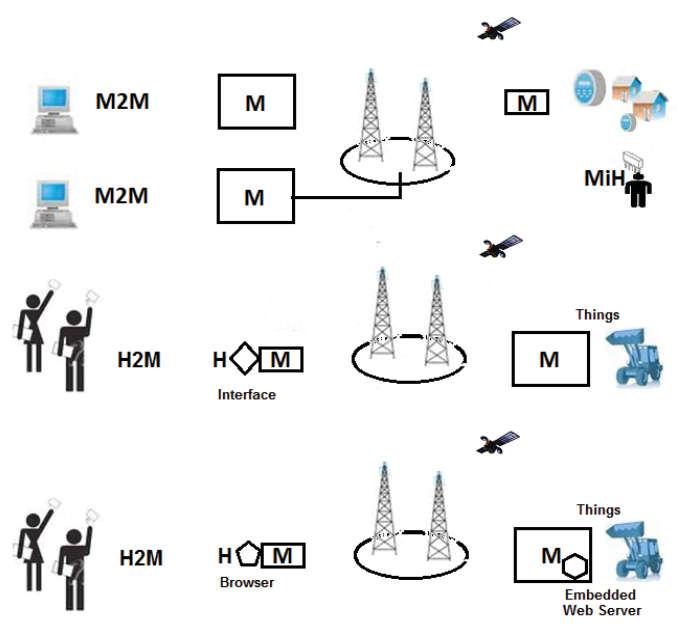
Intuitively, an M2M/H2M environment comprises three basic elements:
- The data integration point (DIP);
- The communication network;
- The data end point (DEP) (again, a Machine M) (see Figure 5, where the process (X) and application (Y) form the actual functional end points).
Typically, a DEP refers to a microcomputer system, one end of which is connected to a process or to a higher level subsystem via special interfaces; the other end is connected to a communication network. However, the DEP can also be a Machine M in a Human H, as is the case in the MiH environment. Many applications have a large base of dispersed DEPs. A DIP can be an Internet server, a software application running on a firm-resident the host, or an application implemented as a cloud service. Basic applications include, but are not limited to, smart meters, e-health, track-and-trace, monitoring, transaction, control, home automation, city automation, connected consumers, and automotive.
As noted earlier, at a macro level, an IoT comprises a remote set of sensing assets (Sensing Domain, also known as M2M Domain in a M2M environment), a Network Domain, and an Applications Domain. Figure 6 provides illustrative pictorial view of the domains.
A High-Level M2M System Architecture (HLSA) (Figure 7) is defined in the ETSI TS 102 690 V1.1.1 (2011-10) specification that is useful to the present discussion. The HSLA comprises the Device and Gateway Domain, the Network Domain, and the Applications Domain.
The Device and Gateway Domain is composed of the following elements:
1 M2M Device. A device that runs M2M Application(s) using M2M Service Capabilities. M2M Devices connect to Network Domain in the following processes:
- Case 1 “Direct Connectivity”. M2M Devices connect to the Network Domain via the Access network. The M2M Device performs the procedures.
- Case 2 “Gateway as a Network Proxy”. The M2M Device connects to the Network Domain via an M2M Gateway. M2M Devices connect to the M2M Gateway using the M2M Area Network. The M2M Gateway acts as a proxy for the Network Domain toward the M2M Devices that are connected to it. Examples of procedures that are proxied include authentication, authorization, management, and provisioning. (M2M Devices may be connected to the Networks Domain via multiple M2M Gateways).
2 M2M Area Network. Provides connectivity between M2M Devices and M2M Gateways. Examples of M2M Area Networks include PAN technologies such as IEEE 802.15.1, ZigBee, Bluetooth, IETF ROLL, ISA100.11a, among others, or local networks such as PLC, M-BUS, Wireless M-BUS, and KNX. KNX (administered by the KNX Association) is an OSI-based network communications protocol for intelligent buildings defined in standards CEN EN 50090 and ISO/IEC 14543) (KNX is the follow-on standard built on the European Home Systems Protocol (EHS), BatiBUS, and the European Installation Bus (EIB or Instabus). Effectively, KNX uses the communication stack of EIB but augmented with the physical layers and configuration modes BatiBUS and EHS; thus, KNX includes the following PHYs: Twisted pair wiring (inherited from the BatiBUS and EIB Instabus standards). This approach uses differential signaling with a signaling speed of 9,6 kbps. Media access control is controlled with the CSMA/CA method; Powerline networking (inherited from EIB and EHS); Radio (KNX-RF); Infrared; and Ethernet (also known as EIBnet/IP or KNXnet/IP).x
3 M2M Gateway: A gateway that runs M2M Application(s) using M2M Service Capabilities. The Gateway acts as a proxy between M2M Devices and the Network Domain. The M2M Gateway may provide service to other devices (e. g., legacy devices) connected to it those are hidden from the Network Domain. As an example, an M2M Gateway may run an application that collects and treats various information (e. g., from sensors and contextual parameters).
The Network Domain is composed of the following elements:
1 Access Network. A network that allows the M2M Device and Gateway Domain to communicate with the Core Network (CoN). Access Networks include (but are not limited to) xDSL (Digital Subscriber Line), HFC (Hybrid Fiber Coax), satellite, GERAN (GSM/EDGE Radio Access Network), UTRAN (UMTS Terrestrial Radio Access Network), eUTRAN (evolved UMTS Terrestrial Radio Access Network), WLAN, and WiMAX (Worldwide Interoperability for Microwave Access).
2 Core Network. A network that provides the following capabilities (different CoNs offer different feature sets):
- IP connectivity at a minimum, and possibly other connectivity means;
- Service and network control functions;
- Interconnection (with other networks);
- Roaming;
- CoNs include (but are not limited to) 3GPP CoNs, ETSI TISPAN CoN, and 3GPP2 CoN.
3 M2M Service Capabilities:
- Provide M2M functions that are to be shared by different applications;
- Expose functions through a set of open interfaces;
- Use CoN functionalities;
Simplify and optimize application development and deployment through hiding of network specificities.
The “M2M Service Capabilities” along with the “CoN” is known collectively as the “M2M Core.” The Applications Domain is composed of the following elements:
1 M2M applications. Applications that run the service logic and use M2M Service Capabilities accessible via an open interface.
There are also management functions within an overall M2M Service Provider Domain, as follows:
1 Network Management Functions. Consists of all the functions required to manage the Access and CoNs; these functions include provisioning, supervision, and fault management.
2 M2M Management Functions. Consists of all the functions required to manage M2M Service Capabilities in the Network Domain. The management of the M2M Devices and Gateways uses a specific M2M Service Capability.
- The set of M2M Management Functions include a function for M2M Service Bootstrap. This function is called M2M Service Bootstrap Function (MSBF) and is realized within an appropriate server. The role of MSBF is to facilitate the bootstrapping of permanent M2M service layer security credentials in the M2M Device (or M2M Gateway) and the M2M Service Capabilities in the Network Domain.
- Permanent security credentials that are bootstrapped using MSBF are stored in a safe location, which is called M2M Authentication Server (MAS). Such a server can be an AAA server. MSBF can be included within MAS, or may communicate the bootstrapped security credentials to MAS, through an appropriate interface (e.g., the DIAMETER protocol defined in IETF RFC 3588) for the case where MAS is an AAA server).
The H2M portion of the IoT could theoretically make use of these same mechanisms and capabilities, but the information flow would likely need to be front-ended by an access layer (which can also be seen as an application in the sense described above) that allows the human user to interact with the machine using an intuitive interface. One such mechanism can be an HTML/HTTP-based browser that interacts with a suitable software peer in the machine (naturally this requires some higher level capabilities to be supported by the DEP/machine in order to be able to run an embedded web server software module.) When used in embedded devices or applications, web servers must assume that they are secondary to the essential functions the device or application must perform; as such, the web server must minimize its resource demands and should be deterministic in the load it places on a system. As an illustrative example of an embedded web server, Oracle’s GoAhead WebServer is a simple, portable, and compact web server for embedded devices and applications; it runs on dozens of operating environments and can be easily ported and adapted. The GoAhead WebServer is a simple, compact web server that has been widely ported to many embedded operating systems. Appweb is faster and more powerful – but requires more memory. If a device requires a simple, low-end web server and has little memory available, the GoAhead WebServer is ideal; if the device needs higher performance and extended security, then App-web is the right choice. As one of the most widely deployed embedded web servers, Appweb is being used in networking equipment, telephony, mobile devices, consumer and office equipment as well as hosting for enterprise web applications and frameworks. It is embedded in hundreds of millions of devices. The server runs equally well stand-alone or in a web farm behind a reverse proxy such as Apache.x
M2M Applications Examples and Satellite Support
This section looks at some IoT/M2M applications, in general, and then focuses on satellite-based support of the space.
Related to IoT applications, proponents make the observation that:
Examples of General Applications
This section provides a sample of applications than can be provided with/by IoT, although any such survey is invariably incomplete and is limited in the temporal domain (with new applications being added on an ongoing basis). We look at applications that are already emerging and/or have a lot of current industry interest. Typically, in a general context, IoT applications range widely from energy efficiency to logistics and from appliance control to “smart” electric grids. Indeed, there is an increasing interest in connecting and controlling, in real time, all sorts of devices for personal healthcare (patient monitoring fitness monitoring), building automation [also known as building automation and control (BA&C) – for example, security devices/cameras; Heating, Ventilation, and Air-Conditioning (HVAC); AMRs]; residential/commercial control (e. g., security HVAC, lighting control, access control, lawn, and garden irrigation); consumer electronics (e. g., TV, DVRs); PC and peripherals (e. g., mouse, keyboard, joystick, wearable computers); industrial control (e. g., asset management, process control, environmental, energy management); and supermarket/supply chain management (this being just a partial list).
Vertical industries in arenas such as automotive and fleet management, telehealth (also called telecare by some) and Mobile Health (m-Health – when mobile communications are used), energy and utilities, public infrastructure, telecommunications, security and defense, consumer telematics, Automated Teller Machines (ATMs)/kiosk/POS, and digital signage are in the process deploying IoT services and capabilities. Proponents make the claim that IoT will usher in a wide range of smart applications and services to cope with many of the challenges individuals and organizations face in their everyday lives. For example, remote healthcare monitoring systems could aid in managing costs and alleviating the shortage of healthcare personnel; intelligent transportation systems (ITSs) could aid in reducing traffic congestion and the issues caused by congestion such as air pollution; smart distribution systems from utility grids to supply chains could aid in improving the quality and reducing the cost of their respective goods and services; and tagged objects could result in more systematic recycling and effective waste disposal. These applications may change the way societies function and, thus, have a major impact on many aspects of people’s lives in the years to come. Many of today’s home entertainment and monitoring systems often offer a web interface to the end-user; the IoT aims at greatly extending those capabilities to many other devices and many other applications.
A list of (early) applications includes the following (also see Table 3).
Table 3. The Scope of IoT | |||
---|---|---|---|
Service Sector | Application Group | Location (partial list) | Devices (“Things“) of Interest (partial list) |
Real estate (Industrial) | Commercial/institutional | Office complex, school, retail space, hospitality space, hospital, medical site, airport, stadium | UPS, generator, HVAC, fire and safety (EHS), lighting, security monitoring, security control/access |
Industrial | Factory, processing site, inventory room, clean room, campus | ||
Energy | Supply providers/consumers | Power generation, power transmission, power distribution, energy management, AMI (Advanced Metering Infrastructure) | Turbine, windmills, UPS, batteries, generators, fuel cells |
Alternative energy systems | Solar systems, wind system, cogeneration systems | ||
Oil/gas operations | Rigs, well heads, pumps, pipelines, refineries | ||
Consumer and home | Infrastructure | Home wiring/routers, home network access, home energy management | Power systems, HVAC/thermostats, sprinklers, MID, dishwashers, refrigerators, ovens, eReaders, washer/dryers, computers, digital videocameras, meters, lights, computers, game consoles, TVs, PDRs |
Safety | Home fire safety system, home environmental safety system (e. g., CO2), home security/intrusion detection system, home power protection system, remote telemetry/video into home, oversight of home children, oversight of home-based babysitters, oversight of home-bound elderly | ||
Environmentals | Home HVAC, home lighting, home sprinklers, home appliance control, home pools and jacuzzis | ||
Entertainment | TVs, PDRs | ||
Healthcare | Care | Hospitals, ERs, mobile POC, clinic, labs, doctor’s office | MRIs, PDAs, implants, surgical equipment, BAN devices, power systems |
In vivo/home | Implants, home monitoring systems, body area networks (BANs) | ||
Research | Diagnostic lab, pharmaceutical research site | ||
Industrial | Resource automation | Mining sites, irrigation sites, agricultural sites, monitored environments (wetlands, woodlands, etc.) | Pumps, valves, vets, conveyors, pipelines, tanks, motors, drives, converters, packaging systems, power systems |
Fluids management | Petrochemical sites, chemical sites, food preparation site, bottling sites, wineries, breweries | ||
Converting operations | Metal processing sites, paper processing sites, rubber/plastic processing sites, metalworking site, electronics assembly site | ||
Distribution | Pipelines, conveyor belts | ||
Transportation | Nonvehicular | Airplanes, trains, buses, ships/boats, ferries | Vehicles, ships, planes, traffic lights, dynamic signage, toll gates, tags |
Vehicles | Consumer and commercial vehicle (car, motorcycle etc.), construction vehicle (e. g., crane) | ||
Transportation subsystems | Toll booths, traffic lights and traffic management, navigation signs, bridge/tunnel status sensors | ||
Retail | Stores | Supermarkets, shopping centers, small stores, distribution centres | POS terminals, cash registers, vending machines, ATMs, parking meters |
Hospitality | Hotel, restaurants, cafe, banquet halls, shopping malls | ||
Speciality | Banks, gas stations, bowling, movie theaters | ||
Public safety and security | Surveillance | Radars, military security, speed monitoring systems, security monitoring systems | Vehicles, ferries, subway trains, helicopters, airplanes, video cameras, ambulances, police cars, fire trucks, chemical/radiological monitors, triangulation systems, UAVs |
Equipment | Vehicles, ferries, subway trains, helicopters, airplanes | ||
Tracking | Commercial trucks, postal trucks, ambulances, police cars | ||
Public infrastructure | Water treatment sites, sewer systems, bridges, tunnels | ||
Emergency services | First responders | ||
IT systems and networks | Public networks | Network facilities, central offices, data centers, submarine cable, cable TV headends, telco hotels, cellular towers, poles, teleports, ISP centers, lights-off sites, NOCs | Network elements, switches, core routers, antenna towers, poles, servers, power systems, backup generators |
Enterprise networks | Data centers, network equipment (e. g. routers) |
- Things on the move:
- Retail;
- Logistics;
- Pharmaceutical;
- Food;
- Ubiquitous intelligent devices:
- Smart appliances;
- Efficient appliances via the use of eco-aware/ambient-aware things;
- Interaction of physical and virtual worlds; executable tags, intelligent tags, autonomous tags, collaborative tags;
- Intelligent devices cooperation;
- Ubiquitous readers;
- Ambient and assisted living:
- Health;
- Intelligent home;
- Smart living;
- Security-based living;
- Transportation;
- Education and Information;
- Environmental aspects/resource efficiency:
- Energy and resource conservation;
- Advanced Metering Infrastructure (AMI);
- Energy harvesting (biology, chemistry, induction);
- Power generation in hash environments;
- Energy recycling;
- Pollution and disaster avoidance.
A longer, but far from complete, list of applications includes the following:
- Public Services and Smart Cities:
- Telemetry: for example, smart metering, parking metering, and vending machines;
- ITSs and traffic management;
- Connecting consumer and citizens to public infrastructure (such as public transportation);
- In-building automation, municipal and regional Infrastructure;
- Metropolitan operations (traffic, automatic tolls, fire, and so on);
- Electrical grid management at a global level, SGs;
- Electrical Demand Response (DR) at a global level.
- Automotive, Fleet Management, Asset Tracking:
- e-Vehicle: for example, navigation, road safety, and traffic control;
- Driver safety and Emergency Services;
- Fleet management systems: hired-car monitoring, goods vehicle management;
- Backseat Infotainment device integration;
- Next-gen GPS services;
- Tracking such as asset tracking, cargo tracking, and order tracking.
- Commercial Markets:
- Industrial monitoring and control; for example, industrial machines and elevator monitoring;
- Commercial building and control;
- Process control;
- Maintenance automation;
- Home automation;
- Wireless AMR/Load Management (LM);
- Homeland security applications: chemical, biological, radiological, and nuclear wireless sensors;
- Military sensors;
- Environmental (land, air, sea) and agricultural wireless sensors;
- Finance: POS terminals, ticketing;
- Security: Public surveillance, personal security.
- Embedded Networking Systems in the Smart Home and Smart Office:
- Smart appliances: for example, AC power control, lighting control, heating control, and low-power management;
- Automated home: remote media control;
- Smart meters and energy efficiency: efficiencies obtained by exploiting the potential of theSG;
- Telehealth (e-health): Assisted living and in-home m-health services (including remote monitoring, remote diagnostic);
- Security and emergency services: integrated remote services.
Figure 8 depicts a grouping of applications, particularly in the M2M context.
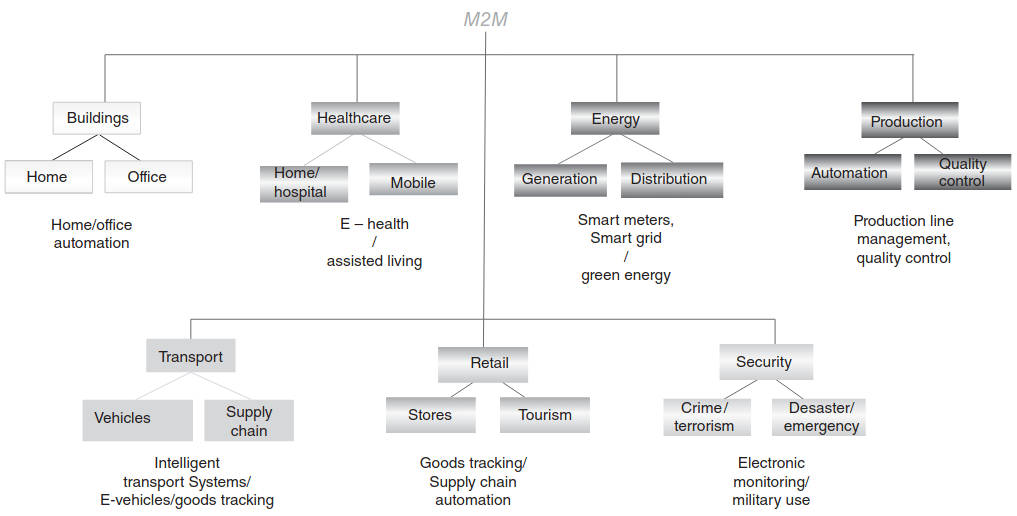
As should be clear by now, some of the possible short-term applications include the following:
- Building automation and remote control (facilitating efficient commercial spaces);
- SE (supporting office building/home energy management);
- Healthcare (providing health and fitness monitoring);
- Home automation (giving rise to smart homes);
- Retail services (enabling smart shopping).
Table 4 provides some examples by category as defined in 3GPP Machine-Type Communications (MTCs) documentation (MTC is the term used in 3GPP to describe M2M systems).
Table 4. Examples of MTC Applications as Defined in 3GPP TS 22.368 Release 10 | |
---|---|
Category | Specific Example |
Consumer devices | Digital camera Digital photo frame eBook |
Health monitoring vital signs | Remote diagnostics Supporting the aged or handicapped Web access telemedicine points |
Metering | Gas Grid control Heating Industrial metering Power Water |
Payment | Gaming machines Point of sales Vending machines |
Remote maintenance/control sensors | Elevator control Lighting Pumps Valves Vehicle diagnostics Vending machine control |
Service Area MTC applications | Backup for landline Car/driver security Control of physical access (e. g., to buildings) Security surveillance systems |
Tracking and tracing fleet management | Asset tracking Navigation Order Management Pay as you drive Road tolling Road traffic optimization/steering Traffic information |
In recent years, ETSI has published a number of use cases for IoT (specifically for M2M) applications in the following documents:
- ETSI TR 102 691: “Machine-to-Machine communications (M2M); Smart Metering Use Cases.”
- ETSI TR 102 732: “Machine to Machine Communications (M2M); Use cases of M2M applications for eHealth.”
- ETSI TR 102 897: “Machine to Machine Communications (M2M); Use cases of M2M applications for City Automation.”
- ETSI TR 102 875: “Access, Terminals, Transmission and Multiplexing (ATTM); Study of European requirements for Virtual Noise for ADSL2, ADSL2plus and VDSL2.”
- ETSI TR 102 898: “Machine to Machine Communications (M2M); Use cases of Automotive Applications in M2M capable networks.”
- ETSI TS 102 412: “Smart Cards; Smart Card Platform Requirements Stage 1 (Release 8).”
Some of these (ETSI-covered) applications are discussed in the sections that follow.
City Automation. Some applications in this domain include but are not limited to the following:
- Traffic Flow Management System in combination with Dynamic Traffic Light Control;
- Street Light Control;
- Passenger Information System for Public Transportation;
- Passive surveillance.
Generic city sensors include environmental sensors and activity sensors. Environmental sensors include devices capable of thermal, hygrometric, anemometric, sound, gas, particles light or other EM spectrum, and seismic measurements. Activity sensors include devices capable of pavement/roadway pressure, vehicle and pedestrian detection, and parking space occupancy measurements.
ETSI TR 102 897: “Machine to Machine Communications (M2M); Use cases of M2M applications for City Automation” provides the following description of these applications:
Use Case 1: Traffic Flow Management System in Combination with Dynamic Traffic Light Control. The flow of road traffic within cities depends on a number of factors such as the number of vehicles on the road, the time and the day, the current or expected weather, current traffic issues and accidents, as well as road construction work. Traffic flow sensors provide key traffic flow information to a central traffic flow management system; the traffic flow management system can develop a real-time traffic optimization strategy, and, thus, endeavor to control the traffic flow. The traffic control can be achieved by dynamic information displays informing the driver about traffic jams and congested roads; traffic signs can direct the traffic to utilize less used roads. The Traffic Flow Management system can also interact with controllable traffic lights to extend or to reduce the green light period to increase the vehicle throughput on heavily-used roads; dynamically changeable traffic signs can lead to an environment where the vehicular traffic is managed more efficiently, thus enabling cities to reduce fuel consumption, air pollution, congestions, and the time spent on the road.
Use Case 2: Street Light Control. Street lights are not required to shine at the same intensity to accomplish the intended safety goal. The intensity may depend on conditions such as moonlight or weather. Adjusting the intensity helps to reduce the energy consumption and the expenditures incurred by a municipality. The street light controller of each street light segment is connected (often wirelessly) with the central street light managing and control system. Based on local information measured by local sensors, the control system can dim the corresponding street lights of a segment remotely or is able to switch street lights on and off.
Use Case 3: Passenger Information System for Public Transportation. Public transportation vehicles, such as buses, subways, and commuter trains operate on a schedule that may be impacted by external variables and, thus, have a degree of variability compared with a baseline formal schedule. Passengers need to know when their next connection is available; this information also allows passengers to select alternative connections in the case of longer delays. In this application, the current locations of the various public transport vehicles are provided to the central system that is able to match the current location with the forecasted location at each time or at specific check points. Based on the time difference, the system is able to calculate the current delay and the expected arrival time at the upcoming stops. The vehicle location can be captured via check points on the regular track or via GPS/GPRS tracking devices that provide the position information in regular intervals. Two approaches are possible:
- With a Check Point based approach, the line number (of the bus or the street car) is captured at each station where the vehicle stops regularly, or at defined check point in between. Because of the fact that the sensor at a specific station is able to provide the data to the central system, the expected delay can be calculated by comparing the information of the scheduled arrival time and the actual arrival time. This change can be added to the arrival time displayed at each following station. Each vehicle must be equipped with a transponder (variously based on infrared, RFID, short-range communication, or optical recognition). In addition, each station has to be equipped with one or more check point systems that are able to readout or to receive the line number information of the vehicle. In case of larger stations with several platforms, multiple systems are needed.
- With a GPS/GPRS-based approach, each vehicle has to be equipped with a GPS/GPRS tracking device that provides beside the current position also the information that can be directly or indirectly matched to the serviced line number. Based on the “regular” position/time pattern, the system is able to calculate the actual time difference and provide the expected time on the passenger display.
A combination of check point based and GPS/GPRS-based solution can be used to integrate railed vehicles (such as subways and street cars) and road vehicles (such as buses).
Automotive Applications. IoT/M2M automotive and transportation applications focus on safety, security, connected navigation, and other vehicle services such as, but not limited to, insurance or road pricing, emergency assistance, fleet management, electric car charging management, and traffic optimization. These applications typically entail IoT/M2M communication modules that are embedded into the car or the transportation equipment. Some of the technical challenges relate to mobility management and environmental hardware considerations.
bCall (Breakdown Call). A bCall sends the current vehicle position to a roadside assistance organization and initiates a voice call. The bCall trigger is usually a switch that is manually pushed by the user in order to activate the service. An “enhanced” bCall service allows current vehicle diagnostic information to be transmitted in addition to the vehicle position.
Stolen Vehicle Tracking. A basic application for automotive M2M communications is tracking of mobile assets – either for purposes of managing a fleet of vehicles or to determine the location of stolen property. The goal of a Stolen Vehicle Tracking (SVT) system is to facilitate the recovery of a vehicle in case of theft. The SVT service provider periodically requests location data from the Telematics Control Unit (TCU) in the vehicle and interacts with the police. The TCU may also be capable of sending out automatic theft alerts based on vehicle intrusion or illegal movement. The TCU may also be linked to the Engine Management System (EMS) to enable immobilization or speed degradation by remote command. Vehicles contain embedded M2M devices that can interface with location determination technology and can communicate via a mobile cellular network to an entity (server) in the M2M core. The M2M devices will communicate directly with the telecommunications network; the M2M devices will interface with location-determination technology such as stand-alone GPS, or network-based mechanisms such as assisted GPS, Cell ID, and so on. For theft-tracking applications, the M2M device is typically embedded in an inaccessible or inconspicuous place so that it may not be easily disabled by a thief. The Tracking Server is an entity located in the M2M core and owned or operated by the asset owner or service provider to receive, process, and render location and velocity information provided by the deployed assets. The Tracking Server may trigger a particular M2M device to provide a location/velocity update, or the M2M devices may be configured to autonomously provide updates on a schedule or upon an event-based trigger.
Remote Diagnostics. Remote diagnostic services can broadly be grouped into the following categories:
- Maintenance minder – when the vehicle reaches a certain mileage (e. g., 90 % of the manufacturer’s recommended service interval since the previous service), the TCU sends a message to the owner or the owner’s named dealership, advising the owner (or the dealership) that the vehicle is due for service.
- Health check – either on a periodic basis or triggered by a request from the owner, the TCU compiles the vehicle’s general status using inbuilt diagnostic reporting functions, and transmits a diagnostic report to the owner, the owner’s preferred dealership, or to the vehicle manufacturer.
- Fault triggered – when a fault [a Diagnostic Trouble Code (DTC)] is detected with one of the vehicle systems, this triggers the TCU to send the DTC code and any related information to the owner’s preferred dealer, or to the vehicle manufacturer.
- Enhanced bCall – when a manual breakdown call is initiated by the owner, the TCU sends both position data and DTC status information to the roadside assistance service or to the vehicle manufacturer.
Fleet Management. The fleet owner wishes to track the vehicles – that is, to know, over time, the location and velocity of each vehicle – in order to plan and optimize business operations. A fleet management application assumes that a fleet of vehicles has been deployed with M2M devices installed that are able to:
- Interface with sensors on the vehicle that measure velocity;
- Interface with devices that can detect position;
- Establish a link with a mobile telecommunication network using appropriate network access credentials, such as a USIM (Universal Subscriber Identity Module).
A server in the fleet owner’s employ receives, aggregates, and processes the tracking data from the fleet and provides this information to the fleet owner. Devices could be configured to autonomously establish communication with the Server via a cellular network either at regular intervals, at prescheduled times, or based on some event such as crossing a geographic threshold. Alternatively, the M2M devices could be commanded by the M2M Server to report their location/velocity data (see Figure 9 for an illustrative example).
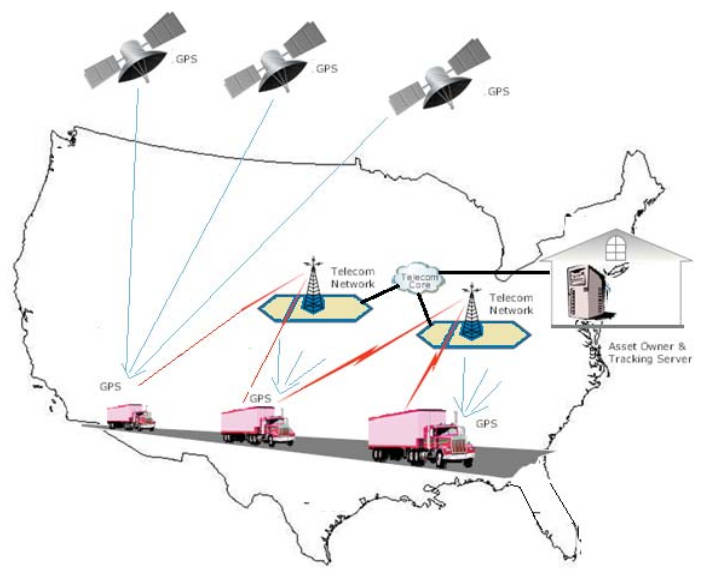
Vehicle-to-Infrastructure Communications. A European Intelligent Transport Systems Directive A directive is a legislative act of the European Union requiring member states to achieve a stated result but without mandating the means of achieving that result.x seeks the implementation of eSafety applications in vehicles. Some vehicle manufacturers have begun to deploy vehicle-to-vehicle communication, for example, in the context of Wireless Access in Vehicular Environments (WAVEs). On the other hand, vehicle to roadside applications are less well developed; in this case, vehicles have embedded M2M devices that can interface with location-determination technology and can communicate via a mobile telecommunications network to an entity (server). This application assumes that vehicles have been deployed with M2M devices installed that are able to:
- Interface with sensors on the vehicle that measure velocity, external impacts;
- Interface with devices that can detect position;
- Establish a link with a mobile telecommunication network using appropriate network access credentials, such as a USIM;
- Upload or download traffic and safety information to a Traffic Information Server.
Devices could be configured to establish communication with the Server via the cellular network based on some event triggered by a vehicle sensor such as external impact, motor failure, and so on. For example, the traffic information server pushes roadside or emergency information out to vehicles based on location (cell location or actual location). Or, vehicle information is pushed to the traffic information server based on external sensor information, internal sensor information, or subscription basis.
Insurance Services. Pay-As-You-Drive (PAYD) schemes offer insurers the opportunity to reduce costs based on actual risk, and provide more competitive products to the end-user based on getting feedback from the vehicle as to when, where, how, or how far the vehicle is being driven (or a combination of these factors).
Satellite Roles, Context, and Applications
As noted, the M2M satellite applications to date have gravitated to MSS services and to the L-band [some also call these satellite applications as LDR (Low Data Rate) applications]. The satellite industry has referred to many of the MSS-based data communication applications as being M2M applications; while we can accept that description, we tend to look at M2M applications as being more “futuristic” in the sense of being more related to IoT-inspired applications, such as those described earlier. We realize that the revenue stream for the satellite operators is now more in the categories we first introduced in “A General Overview of the Internet of Things and M2M” rather than those in “M2M Applications Examples and Satellite Support“, but as time goes by these other applications may come into play.
Satellite service providers perceive M2M communications as an approach to the global demand for uninterrupted and seamless data connectivity across a mixture of urban, suburban, exurban, rural, and oceanic environments: satellite-based M2M can facilitate the delivery of relatively small quantities of information to and from anywhere in the world (consistent with the requirements of Table 2). Applications include civil government, environmental monitoring and climate analysis, Police and Coast Guard, off-shore oil drilling, and mining. Many other examples of IoT applications can be cited and many more will evolve in the future. For example, SCADA applications are now also being extended to be supported over satellite links. Some propose satellite-based M2M as a way to achieve “Global SCADA.”
Some M2M services offered are only one-way communication; others services support two-way communications; clearly two-way communication is a lot more flexible allowing data collection and monitoring of customer assets. Companies that utilize remote locations, such as oil and gas wells, mining sites, water management systems, environmental/weather sites, pollution detection systems, early warning systems, and remote security will benefit from cost-effective, two-way M2M services for asset monitoring solutions.
Observers are noticing an increased demand for satellite services from several companies associated with finance, energy, and maritime industries. Although at press time satellite-based services were only a small share of the M2M market, which is largely dominated by cellular systems (around 2 % in terms of volume and 6 % of revenue in 2011), M2M is a growing segment for the satellite industry: forecasts say the global satellite M2M market will reach $3 billion by 2016. The region with the highest rate of progress will be the Asia-Pacific with developments in countries such as China, Indonesia, Vietnam, and India. Proponents make the case that “M2M Market represents an interesting and potentially huge revenue stream for the satellite industry with opportunities in many markets, particularly vertical ones”; perhaps the opportunity is “significant” rather than “huge.” Some industry observers see growth opportunities in fixed asset tracking while others see it in mobile asset tracking. Iridium and Globalstar reportedly expect growth in the mobile M2M market, whereas SkyWave and Inmarsat reportedly believe that their future growth would come mainly from fixed asset monitoring.
Figure 10 shows how the solutions from different providers are positioned relative to one another in terms of their optimal amount of data needed to be transferred each month.
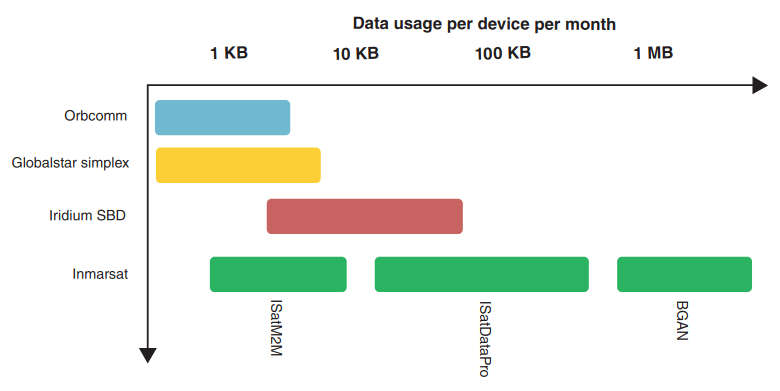
Globalstar and Orbcomm are the most direct competitors at the low end of the market. Iridium has focused on satellite solutions designed to carry 10 KB plus of data each month; the recent introduction of ISatDataPro by Inmarsat now provides an alternative at those usage rates to services by Iridium.
Antennas for Satellite M2M Applications
M2M Antenna types include the following:
- Embedded Antennas. Most M2M products employ multiband internal antennas (planar inverted F) that are galvanically connected to the radiating ground plane of the product. These antenna elements are tailored to match the radiating properties of the product itself, so the product as a whole radiates efficiently over the frequency bands specified. Each product has unique electromagnetic properties owing to size, shape, and interference.
- Stubby Antennas. These antennas are external to the product but need to be carefully tuned to the electromagnetic requirements of the product in order to achieve optimal performance over the frequency bands specified.
- (Mini) Very Small Aperture Terminal (VSAT) Antennas. These are tracking antennas used for Ku-band and Ka-band that for M2M-related applications (especially in the broadband range) are typically 1 m or smaller.
Figures 11 and 12 depict some typical antennas.
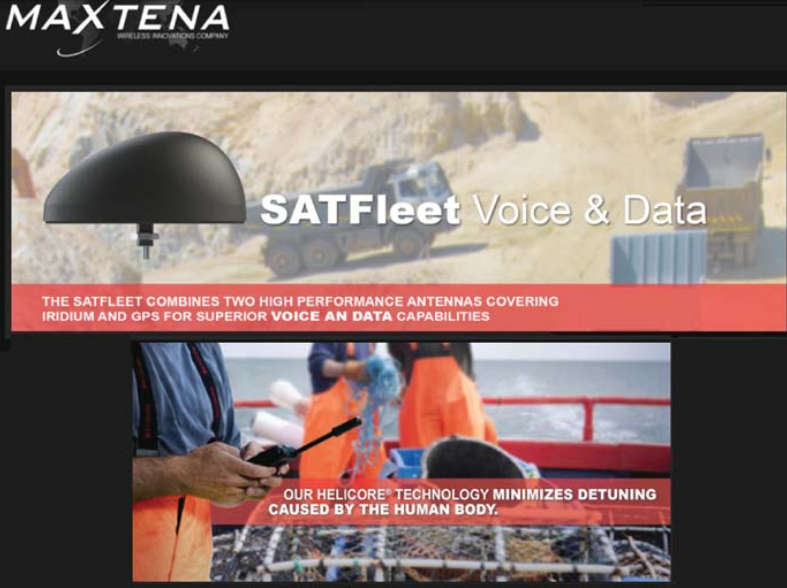
M2M Market Opportunities for Satellite Operators
Earlier we noted that the market can be classified into the following arenas: remote structures such as O&G platforms; land mobile platform include vehicles; maritime platforms; aeronautical platform; and civil infrastructure management. We provide some assessment of these segments below.
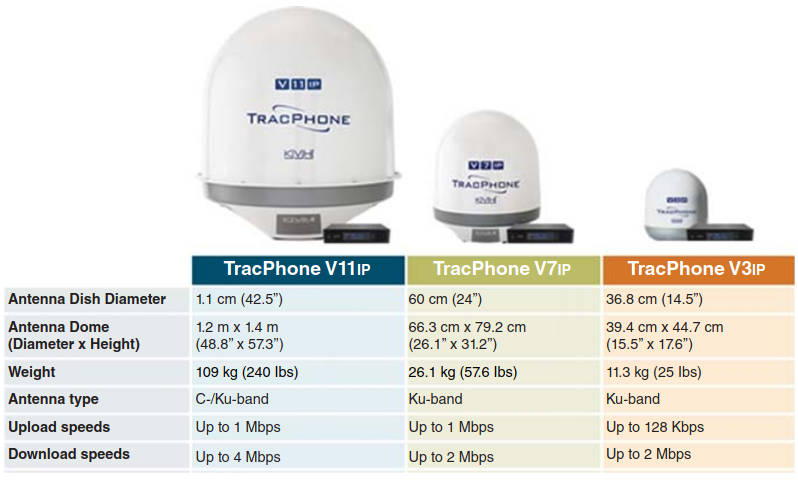
Maritime. In spite of the expected growth of the VSAT systems in the Ku– and Ka-band (as discussed in High Throughput Satellites (HTS) and KA/KU Spot Beam Technologies“Applications and Design Considerations of HTS Satellites” and Aeronautical Mobility Services“Key Technologies and Innovations of Aeronautical Applications”), the MSS operators as an aggregate still account for over 95 % of the maritime satellite communication market (in terms of terminals). All the major MSS operators – Inmarsat, Iridium, Orbcomm, Globalstar, and Thuraya (Dubai – UAE) – serve the maritime market; however, only Inmarsat, Iridium, and Thuraya have a direct focus on the maritime sector. Inmarsat is the maritime/MSS market leader. At present, MSS services, and in particular the legacy Inmarsat services, are widely utilized across the maritime world for a plethora of mission-critical communications. As noticed in Maritime and Other Mobility Services“Emerging Satellite-Based Mobility Applications for Maritime”, at press time about 71 % of the maritime terminals were M2M and safety communications (e. g., Inmarsat-C), 13 % were narrowband terminals (e. g., Inmarsat-B, FleetPhone, Thuraya Seagull), and 16 % were broadband terminals (e. g., FleetBroadband, Iridium OpenPort, Iridium Pilot).
We also noted in Maritime and Other Mobility Services“Emerging Satellite-Based Mobility Applications for Maritime” that merchant ships constitute 65 % of the maritime M2M market:
- government,
- O&G,
- leisure boats,
- passenger ships,
- and fishing ships each comprises about 7 % of the market.
FSS operators tend to focus on the provision of satellite capacity while MSS operators are typically involved in service delivery; in fact, MSS operators provide a menu of services, including medium data rate M2M services and voice/fax, safety-support communications, narrowband data (<128 kbps) services; but they also provide broadband services, such as Inmarsat FleetBroadband and Iridium Pilot. A number of MSS products are compliant with maritime safety communications requirements. The leading two MSS systems, Iridium and Inmarsat, provide global coverage or near-global coverage (global for Iridium, while the Inmarsat system does not cover the polar caps).
M2M communication, in general, and asset-tracking solutions, in particular, are gaining popularity in the maritime sector as the subtending technologies become cheaper and easier to deploy and manage. In addition, an increasing number of value-added services are being offered through low-data-rate terminals, enabling vessel owners to increase safety, security, and operational efficiency. Typical applications include geolocation information, machine surveillance, surveillance of various conditions (e. g., temperature and speed), SCADA, and ship identification services used for Automatic Identification System (AIS) or Long-Range Identification and tracking (LRIT).
Container tracking could, in theory, be an MSS/M2M application; however, ship-board techniques using other (short range) wireless networks are generally needed in addition to one satellite-enabled gateway, since each container does not have direct line-of-sight to the satellite (see Figure 13).
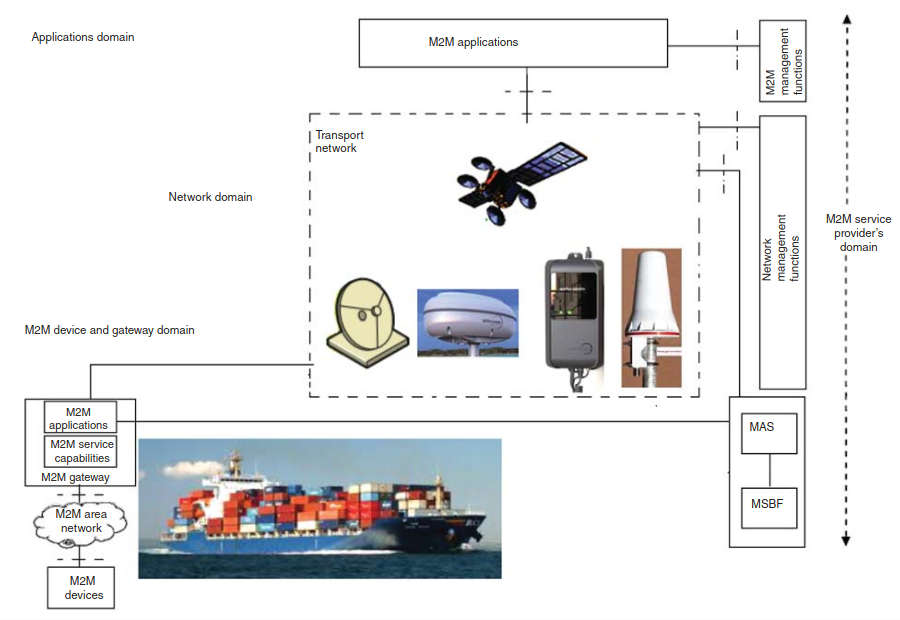
While the majority of the maritime terminals as of press time were still LDR-type terminals used principally for safety communications and also used for other related basic M2M applications, broadband Internet access and streaming video have been the driver of sector growth in recent years; however, evolving IoT/M2M are not limited to low-speed-only applications.
In 2014, there were an estimated 52 000 broadband terminals, 50 000 narrow-band terminals, 260 000 M2M terminals, and 12 500 VSAT terminals in use. LDR terminals (used for regulation and security) represent the bulk of MSS terminals; these systems also support data loggers. While the deployment of MSS broadband services terminals enjoys a growth trajectory that results in an estimated 130 000 terminals in use by 2022, the number of terminals with legacy narrowband service, typically delivered over the Inmarsat-3 constellation, has been declining due to migration to MSS broadband and VSAT services. By 2022, it is estimated that there will be 130 000 broadband terminals, 40 000 narrowband terminals, 480 000 M2M terminals, and 31 000 VSAT terminals in use.
In terms of specific vertical application within the maritime industry cited above (merchant ships, government, O&G, leisure boats, passenger ships, and fishing ships).
- Fishing vessels. The need to control the catches of fishing vessels is driving a number of countries to require the mandatory adoption of electronic logbooks, which in turn requires maritime connectivity.
- Passenger ships. Passenger ships were equipped with an estimated 11 000 satellite terminals at press time. The growth rate for satcom terminals in passenger ships is expected to be relatively low with a CAGR of approximately 4 % over the next few years, since the high-end market is already well-served. Although migrations to a new generation of services, specifically broadband Internet-access Ku/Ka VSAT-based services, still present an opportunity, these substitutions will have relatively limited impact on the growth in terminals counts; however, the revenue potential will grow.
- O&G. Offshore O&G activity encompasses exploration development, production, and decommissioning. Maritime satellite communications usage is particularly intensive in the exploration phase by both survey vessels, mobile rigs, and supporting vessels. For offshore operations away from nearby terrestrial connectivity, VSAT and MSS services continue to make up the predominant networks.
- Government. An increasing number of government users need to survey, track, and monitor their assets, including at sea; as a consequence, the increased use of low-data-rate M2M devices in a government maritime environment to track cargo, to increase safety and situational awareness, and to support logistics is seen.
Land Mobile Land-mobile low-data-rate services in the M2M space, including asset tracking and fleet management application, are expected to be utilized by about 2,5 million units by 2022 (from a base of about 1,5 million in 2014, with a CAGR of about 7 %, under some high-growth scenario assumptions – but not unreasonable assumptions). It represents the largest market for mobility and has the largest number of deployed systems. It is comprised of commercial and military vehicles, and emergency response vehicles. Retail revenues were forecast to grow from about $2,2B to about $3,5B in 2022 (with a CAGR of 6 %) and wholesale revenues to grow from about $09B to $1,5B. Retail revenues include retail equipment revenues (8 %) and retail services revenues (92 %). Both narrowband and broadband (MSS-based) applications will continue to see deployment. These services support both the communications-on-the-move (COTM) and communications-on-the-pause (COPT), for example, when the land mobile devices become stationary (say overnight). Service capabilities include portable units that provide VoIP and video (for the broadband service options); other capabilities include personal locator beacons and location-based services.
Read also: DVB-S2 Modulation Extensions and Other Advances
This market is typically served by low earth orbit (LEO) operators such as Iridium (with the 9602 transceiver) and Globalstar (with the SPOT service for emergency calls from remote locations, as well as by Inmarsat ISAT M2M providers (e. g., Skywave). It is generally recognized that cellular 3G/LTE services offer competitive alternatives to the satellite market. In 2012, Inmarsat also launched the BGAN M2M data service for real-time applications (supporting 1–50 MB data transfers per month) for applications such as smart metering, SCADA, remote asset monitoring, meteorological and environmental data collection, telemetry for utilities, O&G platform operators, and government agencies.
For a press time example, the Iridium 9602 SBD transceiver (Figure 14), designed to be integrated into a wireless data application with other host system hardware and software, provides a complete solution for a specific application or vertical market.
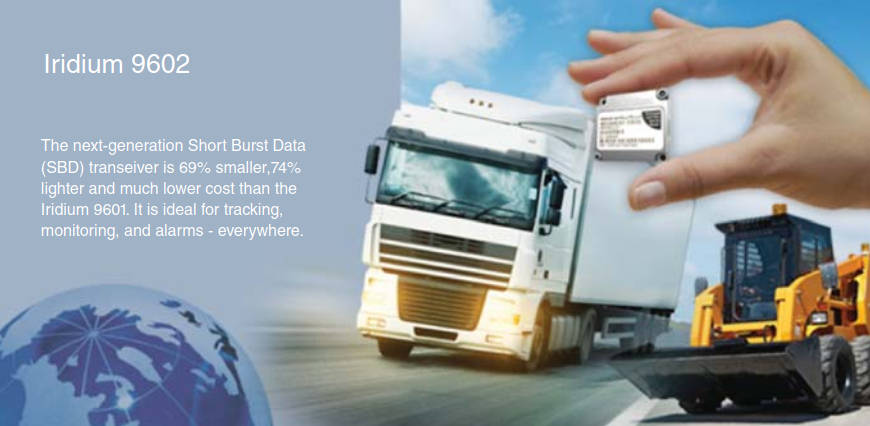
The Iridium 9602 is ideal for M2M solutions, including tracking of maritime vessels, equipment monitoring, and automatic vehicle location. Another typical press time example is the Quake Global’s Quake Q4000 dual-mode satellite/GSM M2M unit (using the Iridium chipset) that allows asset tracking and remote two-way communication for land mobile, maritime, and aeronautical applications (Figure 15).
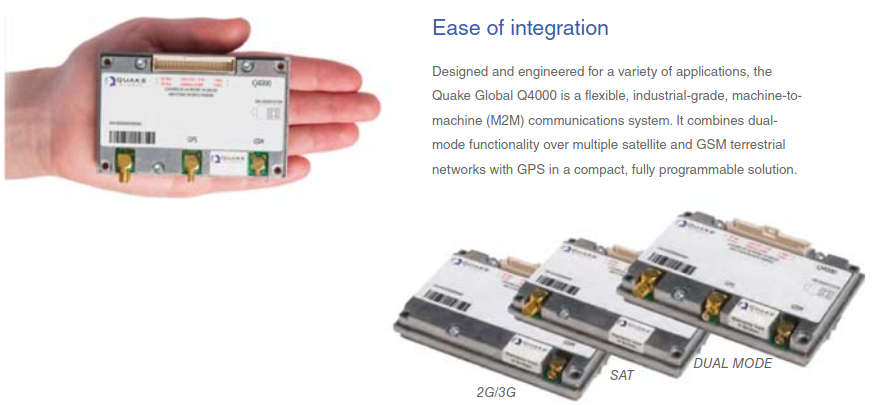
The device supports satellite network (GPS, Iridium, Orbcomm) as well as terrestrial 3G cellular systems; the M2M modems meet the demanding quality requirements found in the heavy equipment and mining industries, among other industries.
Aeronautical. A number of M2M applications exist in the aeronautical space, particularly from companies such as Inmarsat. Having covered aeronautical environments in Aeronautical Mobility Services“Key Technologies and Innovations of Aeronautical Applications”, we only make note of the Automatic Dependent Surveillance-Broadcast (ADS-B) system here.
With ADS-B (and GPS), aircraft can transmit their location and altitude to other nearby aircraft and to air traffic control (ATC). On May 27, 2010, the US Federal Aviation Administration (FAA) issued a final rule mandating ADS-B equipage. Effective on January 1, 2020, any aircraft operating in airspace where a Mode C transponder is required today will also be required to carry an ADS-B OUT transmitter. ADS-B will redefine the paradigm of communications, navigation, and surveillance in Air Traffic Management today.
Already proven in the field and internationally certified as a viable low-cost replacement for conventional radar, ADS-B allows pilots and air traffic controllers to “see” and control aircraft with more precision and over a far larger percentage of the earth’s surface than has ever been possible before. Essentially, ADS-B is a one-for-one replacement for Radar, except that ADS-B is more accurate, reliable, and far more cost effective than Radar. ADS-B capable aircraft use an ordinary GNSS (GPS) receiver to derive their precise position from the GNSS constellation, and then combine that position with any number of aircraft variables, such as speed, heading, altitude, type of aircraft, and flight number. This information is then simultaneously broadcast line-of-sight to ATC ADS-B receivers on the ground and to other aircraft equipped to receive it. A space-based enhancement to ADS-B is an over-the-horizon air traffic management system capable of delivering an uncorrupted ADS-B digital message every second from virtually any ADS-B avionics system to ATC. In essence, this extends ADS-B into areas where a conventional line-of-sight connection between the aircraft and a terrestrial ADS-B ground station is either impossible or impractical to maintain. When an ADS-B equipped aircraft is over the horizon from an ADS-B ground station, an MSS constellation provides an alternate delivery path for the ADS-B signal.
Features include:
- Automatic – “Always ON” – No operator attention is required;
- Dependent – Relies on very accurate GNSS position data;
- Surveillance – Provides aircraft position, altitude, speed, heading, identification, and other data;
- Broadcast – Requires no interrogation or triggering by other stations – data are broadcast to any aircraft or ground station equipped to receive the data link signal.
One example is the utilization of the Globalstar Constellation; the Globalstar’s system is called ADS-B Link Augmentation System (ALAS) by the firm.
Utility Market. Although the near-term outlook for M2M satellite communications solutions is low in the utility market, a demand exists in both developed and developing markets. The developed markets in Europe and North America have seen some deployments of the services, but in the future Latin America and Asia hold potential. With high natural gas prices, abundant renewable energy sources such as hydroelectric power, and in the case of China, widespread air pollution, an ongoing emphasis on distributed generation sources and grid optimization efforts is almost certain. The market is still dominated by MSS forms factors that offer easy satellite and terrestrial integration into small footprints. From a sizing perspective the market for utility satellite communications services remains a market for in-service units, rather than service revenues for bandwidth demand (see Figure 16).

Although new applications continue to emerge across the industry – from remote video monitoring to supporting remote workers in semi-manned substations or generating locations – overall bandwidth needs remain minimal. As such, the introduction of GEO-HTS offerings will find their niche in supporting OA applications and M2M centric services taking a distant second place.
Key Satellite Industry Players and Approaches
This section provides some information on some of the major suppliers for illustrative purposes; this discussion is not intended to be exhaustive in any manner.
Inmarsat. Inmarsat is the maritime/MSS market leader; it has a long history and experience in maritime communications. Inmarsat has been able to maintain its leading position among MSS operators in the maritime segment due to:
- Limited competition for deep-sea communications in the 1990s;
- The company’s globally established service provision and distribution network, and;
- Its continuous product innovations.
Inmarsat M & B: Understanding the System and its ServicesInmarsat’s maritime products include Inmarsat-B, Inmarsat-C, Inmarsat-M, Inmarsat Fleet, and Inmarsat FleetBroadband. Related specifically to M2M, services include Inmarsat D+, IsatM2M, ISatData Pro (developed by Sky-Wave), and BGAN (Broadband Global Area Network) M2M. Two of the leading Inmarsat system-based M2M service providers are SkyWave and EMS Global Tracking. Among all Inmarsat-related maritime businesses, it has been estimated that the operator accounted for more than 70 % of MSS maritime terminals and more than 80 % of MSS maritime wholesale revenue in 2011.
Inmarsat is also pursuing asset monitoring applications, such as SG applications (on the other hand, Iridium, Globalstar, and Orbcomm reportedly see little demand for fixed asset monitoring and SCADA-type applications – their growth to be almost entirely driven by mobile assets, particularly in the transportation segment). In the US, utilities (which are one of the most obvious opportunities for fixed asset monitoring) are governed by rate-of-return legislation that (effectively) encourages investing in capital equipment, giving them an incentive to invest in unlicensed wireless networks, or even wired solutions, even if these are more expensive, rather than incurring ongoing costs for data transmission over cellular or satellite network. In contrast, Inmarsat’s business is more global, and Inmarsat is focused on growth opportunities in BRICA (Brazil, Russia, India, China, Africa) countries, although few MSS low-data-rate solutions are used in at present, and thus it may take time to grow awareness.
Key applicable Inmarsat services include the following (according to the company), listed here for illustrative purposes:
- BGAN M2M. Two-way global IP data service for long-term monitoring and control of fixed assets in unmanned, remote locations.
- IsatM2M. A worldwide two-way, low-data-rate messaging service for tracking, monitoring, and control of remote assets.
- IsatData Pro. Two-way, short-burst data exchange for tracking and monitoring fixed or mobile assets globally.
BGAN M2M is a reliable, global, two-way IP data service designed for long-term M2M management of fixed assets. It connects monitoring and control applications in remote, unmanned locations, giving the user full visibility and management of the dispersed assets across an entire operational area. BGAN M2M is targeted to customers with data volume requirements ranging from megabytes to gigabytes, such as real-time surveillance or high-volume metering and telemetry. It is a 3G satellite network service and provides full IP data connectivity supported by remote terminal management, debugging, and configuration options.
Using robust and lightweight hardware, BGAN M2M enables a wide range of M2M applications. Some features are as follows:
- Global Coverage. BGAN M2M is accessible across the globe except in the extreme polar regions.
- Performance and Latency. Send data using BGAN Standard IP at a rate of up to 448 kbps with a low latency from 800 ms, assuring real-time visibility of critical data.
- Easily Integrated. Simple for field teams to set up and integrate with specific applications, and to maintain without technical expertise or training.
- Robust Terminals. BGAN M2M is accessed through a robust terminal that can be housed in a sturdy, weatherproof unit for long-term unmanned deployment.
- Reliable Network. Operates over our global satellite and ground network, with 99,9 % availability and an operational lifespan expected well into the 2020s.
- Affordable low hardware costs with subscription-based price plans, no minimum connection fee and minimum billing increments.
- Specific applications:
- IP SCADA for data backhaul;
- Asset tracking;
- Fixed monitoring – remote surveillance;
- Fixed monitoring – remote telemetry;
- Fixed monitoring – remote tracking;
- Friendly force tracking;
- Mobile monitoring – remote surveillance;
- Mobile monitoring – remote telemetry;
- Mobile monitoring – remote tracking;
- Oil well head telemetry and monitoring;
- Railway track and crossings;
- Remote control of assets;
- Remote personnel tracking;
- Road signs;
- Secure and encrypted ATM/PoS solution;
- Smart grid;
- Smart metering;
- Telemetry – SCADA;
- Weather, environmental monitoring.
- General applications:
- ECDIS;
- GPS location data look-up-and-send;
- IP SCADA;
- SCADA;
- Surveillance.
Inmarsat only had a few thousand BGAN terminals deployed at press time, but the expectation was for greater deployment in the future.
As an illustrative example, the Hughes 9502 IP satellite terminal provides reliable connectivity over the Inmarsat BGAN for IP SCADA and M2M applications. The Hughes terminal delivers affordable, global, end-to-end IP data connectivity enabling applications in industry sectors such as environmental monitoring, smart grid, pipeline monitoring, compressor monitoring, well site automation, video surveillance, and out-of-band management to primary site communications. The low power consumption (<1 W idle) of the Hughes 9502 makes it possible to provide end-to-end IP connectivity to sites that are off the grid; this equipment provides end-to-end IP connectivity to power-challenged locations that rely upon solar battery arrays involving sensitive power budgets.
Some features are as follows:
- Satellite transmit frequency: 1 626,5–1 675 MHz;
- Satellite receive frequency: 1 518–1 559 MHz;
- GPS frequency: 1 574,42–1 576,42 MHz;
- IDU weight: <1,5 kg;
- IDU dimensions: 15 cm × 20 cm × 45 cm.
IsatM2M is a global, store-and-forward low-data-rate messaging to and from remote assets for tracking, monitoring, and control operations. The service supports critical applications such as transport vehicle security, industrial equipment monitoring, and marine tracking, giving companies visibility and control of fixed or mobile assets. IsatM2M is a two-way burst messaging service that enables a wide range of M2M applications for tracking and monitoring remote fixed or mobile assets on a global basis – whether on land, at sea, or in the air. This next-generation satellite telematics service is based on Inmarsat D+ and offers fast data forwarding rates, quicker responses to polling requests, and shorter time to first transmission.
Some features are as follows:
- Global Coverage. IsatM2M is available across the globe, apart from the extreme polar regions.
- Performance and Latency. Speeds of 10,5 or 25,5 bytes in the send direction and 100 bytes in the receive direction, with latency typically between 30 and 60 s.
- Specific applications:
- Asset tracking;
- Fixed monitoring – remote surveillance;
- Fixed monitoring – remote telemetry;
- Fixed monitoring – remote tracking;
- Friendly force tracking;
- Mobile monitoring – remote surveillance;
- Mobile monitoring – remote telemetry;
- Mobile monitoring – remote tracking;
- Remote personnel tracking.
- General applications:
- GPS location data look-up-and-send;
- SCADA;
- Short message email;
IsatData Pro is a global two-way short message service for M2M communication. It enables companies to track and monitor their fixed or mobile assets, giving them increased visibility of business operations, enhanced efficiency, and greater safety and security for their assets, cargo, and drivers – while lowering operational costs. It is a low-data-rate service ideal for remote management of fixed assets including tracking and telemetry, IsatData Pro operates in near real-time anywhere in the world. With burst-mode communication and a gateway for store-and-forward messaging, IsatData Pro also offers a convenient web-based portal for adjusting settings. Applications can be run on the terminal to reduce data sent over the air. Suitable for mission-critical applications, it offers a wide range of protocols for data collection. One can send 6 400 bytes and receive 10 000 bytes, with a latency of 15–60 s depending on message size. The service operates over the I–4 global satellite network, with an availability of 99,9 % and expected lifespan into the 2020s.
Iridium Communications Inc. Iridium is major player in the M2M/MSS market, and the company has significant revenue stream from these services. The firm supports the handheld telephony and maritime markets, in addition to many other services and markets. The Iridium constellation consists of 66 active polar-orbit satellites and six additional in-orbit spares that provide global voice and data services to satellite phones and to/from integrated transceivers (the company also has nine ground spares for additional backup support). Iridium owns and operates the constellation and resells equipment and access to its MSS services. The satellites are in LEO, operating at a height of about 500 miles above the earth (Figure 17).
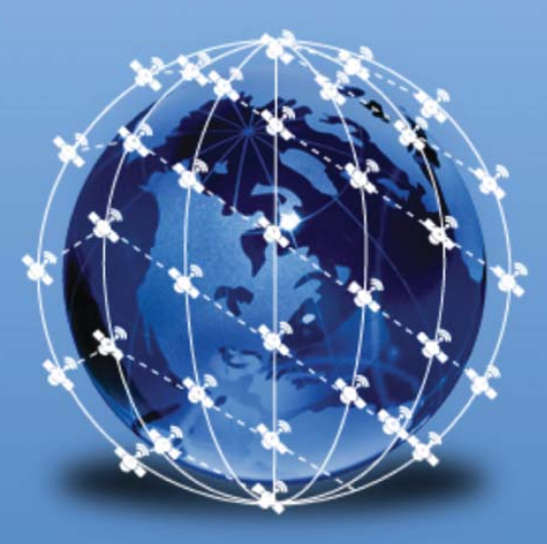
The satellites are “cross-linked” and communicate with neighboring satellites via Ka-band intersatellite links (each satellite can have up to four intersatellite links: two to neighbors fore and aft in the same orbital plane, and two to satellites in neighboring planes to either side). With Iridium NEXT, launched in 2017, Iridium is on schedule to fully replace its current satellite constellation over time and introduce new enhancements and innovations through higher data speeds and increased bandwidth. Iridium M2M will also offer greater transmitting power, able to penetrate buildings and other structures, smaller antennas and transceivers, and lower latency. It will offer backward capability with existing Iridium-connected applications.
A recent listing of the services they offer includes the following (Figure 18):
- Satellite phones;
- Iridium Wi-Fi solutions;
- Iridium Pilot®;
- Modems and modules;
- Broadband services;
- Voice services;
- Data services;
- LRIT (Long-range identification and tracking);
- Tracking and monitoring;
- Personal tracking and monitoring.
Iridium Wi-Fi Solutions. Iridium has developed a suite of Wi-Fi-enabling accessory and software solutions, giving users the ability to use smartphones, tablets, and laptops beyond the reach of existing terrestrial networks by having satellite links behind the locally deployed access points. Combined with your Iridium satellite phone, Iridium AxcessPoint creates a Wi-Fi hotspot, wherever the user is in the world. Usage charges are billed per minute once the Internet connection is established, until the user disconnect your Iridium AxcessPoint.
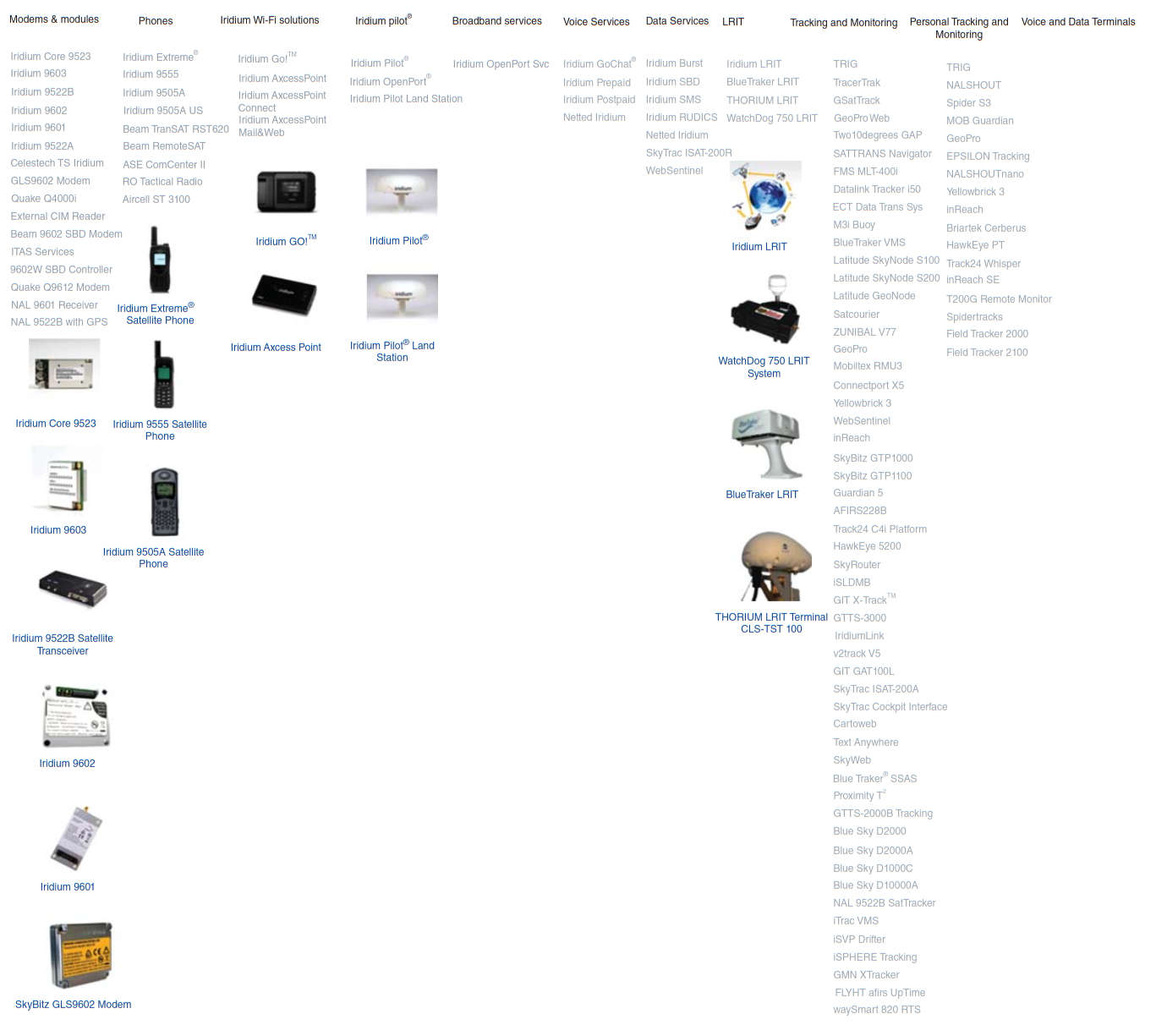
Iridium Pilot®. Iridium provides an integrated antenna, modem, and receiving station to handle broadband data and high-quality voice in one low-cost platform. Options include the following:
- Iridium OpenPort® (being phased out after 2016, and is been replaced by its second generation maritime solution, Iridium Pilot™. Product support will be available until 2016);
- Iridium Pilot;
- Iridium Pilot Land Station.
Iridium Pilot is positioned by the vendor as a next-generation global maritime communications. The outdoor antenna is engineered to perform in blazing sun, frigid cold, or high wind conditions. The ship-borne equipment supported by the Iridium OpenPort broadband is packet switched service that offers highly reliable broadband voice and data communications priced on a number of different pricing options. OpenPort broadband offers the largest and only truly global commercial communication network, providing pole-to-pole coverage for ships at sea, it works everywhere on the planet (for ships traveling in the A4 region, Iridium OpenPort broadband service is the only broadband option available). The system and service can also work as a backup to a larger VSAT service that the ship may have. The linked LEO satellites provide inherent advantages over GEO satellite constellations, supporting low latency links; furthermore, because the Iridium OpenPort broadband service operates in the L-band spectrum, it is mostly impervious to inclement weather. Many customers with mission-critical communications requirements rely on the Iridium OpenPort Service to back-up to VSAT, or other communications on their vessel. The Iridium OpenPort broadband service can substantially reduce the satellite communications costs compared to mini-M, Fleet, or even FleetBroadband, while providing better reliability and throughput. The Iridium OpenPort broadband service offers a wide range of customizable voice and data plans, allowing the users to choose what best suits their needs and their budget.
Some features of the equipment package include the following:
- Antenna:
- Height: 9,06 in. (230 mm);
- Diameter: 22,44 in. (570 mm);
- Weight: 24,25 lb (11 kg).
- Below-Decks Unit:
- Height: 7,78 in. (200 mm);
- Width: 9,84 in. (250 mm);
- Diameter: 2,17 in. (55 mm);
- Weight: 2,98 lb (1,35 kg).
- Data: Up to 134 kbps bidirectional with Iridium OpenPort broadband service.
- Telephony: Three independent phone lines.
- Coverage: Global, pole-to-pole.
Iridium Pilot Land Station is targeted to the land mobile market; also it can be used in fixed environment in remote locations. The equipment is engineered for durability in harsh environments and delivers reliable communications performance and value in a variety of fixed and mobile applications – everywhere on the planet; features include the following:
- Supports data applications such as email, web browsing, or social media;
- Powers up to three simultaneous voice calls, even during data transmission;
- Simply mounts on buildings for quick deployment in remote locations.
Modems and Modules. Iridium has developed an array of transceivers, modems, and receivers that can be integrated by other partners into products they may be developing. For example, available to registered Iridium partners, the Iridium 9522A Satellite Transceiver sends and receives data from equipment anywhere on the planet. It functionally supports all of Iridium’s voice and data services and easily integrates into a wide variety of applications through the RS232 serial interface and AT command set. Some of the products include the Quake Q4000i, the Quake Q9612 Modem, and the SkyBitz GLS9602 Modem.
Data services include the following:
- Iridium Burst;
- Iridium SBD;
- Iridium SMS;
- Iridium RUDICS;
- Netted Iridium®;
- SkyTrac ISAT-200R;
- Latitude WebSentinel.
A brief description of some M2M-relevant services follows.
- Iridium Burst supports a one-to-many global data broadcast service, outbound to remote devices. Delivers data to an unlimited number of enabled devices within a targeted geographic region at a fraction of the cost of comparable services. Iridium Burst service receivers are small, light, and meet many environmental standards;
- Low-cost Short Burst Data (SBD) is an example of an M2M service from Iridium. This service enables user devices to send periodic time-based messages, GPS coordinates, and external sensor data automatically; the use sets up trigger thresholds for the sensors that initiate reports, send alerts, or data transmissions. The service is geared to low-bandwidth applications including telnet sessions. Applications include environmental remote monitoring systems; large animal/herd tracking systems; remote equipment tracking; networks of remote stations for monitoring waste water systems, power network systems, oil delivery systems; and agriculture analysis and monitor system. These systems also allow computer-to-satellite-to-Internet communication, voice communication (e. g., Voice Iridium Privacy Handset), analog voice communication, chat SMS, GPS data acquisition, mail weather blog, and Grab & Go, and sensors connectivity;
- Router-based Unrestricted Digital Internetworking Connectivity Solution (RUDICS) is an example of an M2M service from Iridium. It provides an enhanced gateway termination and origination capability for circuit switched data calls across the Iridium satellite network. When a user places a call to the RUDICS Server located at the Iridium Gateway, the RUDICS Server connects the call to a predefined IP address allowing an end-to-end IP connection between the Host Application and the user;
- The Netted SM Iridium concept combines voice communication and positioning services with less than two-second push-to-talk capability – all in a portable, handheld device. It is currently available only through the Department of Defense’s Iridium gateway, but we believe it has benefits to offer to a wide range of organizations.
LRIT (Long-Range Identification and Tracking) Iridium’s low-latency LRIT solutions provide complete coverage for all seas, including polar sea areas. It allows naval operators to improve maritime safety with tracking, monitoring control, and surveillance of ships, and gather the information needed to maintain optimal operations. Service elements include the following:
- Iridium LRIT;
- BlueTraker LRIT;
- THORIUM LRIT Terminal CLS-TST 100;
- WatchDog 750 LRIT System.
Tracking and Monitoring. Iridium offers an array of tracking and monitoring services and hardware for both “machines” and “people.” From shipping to delivery, these devices are designed to provide organizations with end-to-end tracking. By knowing where items are when they are on the move, asset utilization and productivity are improved and theft/recovery costs are reduced. Figure 18 listed some of the equipment used for these services, and the interested reader should consult the provider’s web site.
These capabilities fit well under the M2M rubric, which is the topic of this chapter.
Iridium M2M. “Iridium M2M” is an integrated capability offered by the firm. Iridium M2M leverages the power of the Iridium’s network to extend the high value of intelligent M2M data flows beyond the barriers of terrestrial networks. The company states that the Iridium satellite network offers truly global M2M service with the lowest latency in the industry – extending the value of intelligent data far beyond the 10 % of the earth serviced by terrestrial networks. With an ecosystem of over 240 partners leveraging Iridium’s core technology to innovate and advance M2M data exchanges, Iridium M2M is the catalyst for cutting-edge solutions across a wide range of markets.
Iridium M2M provides the following:
- 100 % truly global coverage;
- Low latency; near real-time data delivery;
- Higher transmission speeds than terrestrial networks;
- One-price global coverage;
- Low-cost next-generation ultralight transceivers.
As noted earlier, Iridium BurstSM provides cost-effective one-to-many M2M communication. Iridium Burst is the world’s first one-to-many global data broadcast service. Available as an on-demand or dedicated service, Iridium Burst offers secure, simple, cost-effective, and low-latency one-to-many transmission of data to an unlimited number of enabled devices within a targeted geographic region – at a fraction of the cost of comparable services. From command and control of troops and deployed assets to software, firmware and electronic billboard updates, to warnings about tsunamis, fires, earthquakes, tornados, and hurricanes, Iridium Burst transmits highly actionable, customer-specific critical information anywhere on the planet.
Orbcomm. Orbcomm offers M2M products and services, including satellite and cellular connectivity (in conjunction with global network partners), asset tracking and monitoring devices, satellite modems, RFID tags, and web reporting applications. Orbcomm makes use of a cadre of Value Added Resellers (VARs) to place their M2M products into the market, but they are in the process of rationalizing that model through the acquisition of a number of VARs (approximately half-a-dozen in recent years). Orbcomm generate a significant portion of their revenue (approximately 50 %) from sales of wholesale satellite capacity on its own network.
The company launched six next-generation OG2 satellites from Cape Canaveral Air Force Base in Florida aboard a dedicated SpaceX Falcon 9 rocket in June 2014. Eventually, 17 second-generation satellites will be launched to enable the company to deliver adequate service quality. The OG2 satellites are more advanced than its current OG1 satellites and provide existing customers with enhancements, such as faster message delivery, larger message sizes, and better coverage at higher latitudes, while drastically increasing network capacity. In addition, the OG2 satellites are equipped with an AIS payload to receive and report transmissions from AIS-equipped vessels for ship tracking and other maritime navigational and safety efforts, increasing asset visibility and the probability of detection for Orbcomm’s AIS customers.
As an illustrative example, around press time Orbcomm announced that its partner, Savi Technology (Savi), has been awarded a 5-year US Department of Defense contract as the sole provider of the RFID-IV program. The RFID-IV program offers a range of technology solutions, including newly upgraded RFID, satellite-based Enhanced In-Transit Visibility (EITV), and Advanced Intrusion Tracking Detection (ATID) products and services to a wide range of government customers for global asset planning and tracking of personnel, equipment, and sustainment cargo worldwide. In support of the RFID-IV program, Orbcomm and Savi will offer satellite and RFID tags as well as other sensor technologies using Orbcomm’s global communications networks, which will enhance the visibility and security of government cargo in transit. Orbcomm’s tracking and monitoring solutions will provide government customers with the current location of their cargo and send real-time alerts for security breaches and other anomalies such as cargo entering or exiting a geozone outside of predetermined parameters for immediate resolution. US government customers, including the Army, Navy, Air Force, USTRANSCOM, Special Forces, and Defense Logistics Agency, can now seamlessly track and secure their cargo anywhere in the world and access near-real-time operational data through existing government EITV systems or secure, dedicated web portals to meet specific mission needs.
The firm offers a range of devices to track and monitor assets anywhere in the world with complete global coverage. Orbcomm’s dual-mode devices utilize both satellite and cellular communications to manage remote and mobile assets for optimal connectivity, cost, and global coverage (see Table 5 for illustrative examples of M2M devices).
Table 5. Illustrative Examples of M2M Devices (Orbcomm) | |
---|---|
Device | Features |
GT 500 | The ruggedized GT 500 is an environmentally hardened asset tracking solution for the transportation, oil and gas, and other industrial markets. This one-way satellite-based device is fully waterproof and completely self-contained. The GT 500 provides accurate position information and motion detection capabilities for assets worldwide. |
GT 600 | The GT 600 is a self-contained, reliable intrusion detection and tracking solution for the transportation, oil and gas, and other industrial markets. Utilizing one-way satellite communications, the GT 600 supports a security cable that can be used to wrap around container locking bars or other latching mechanisms for maximum cargo security. |
GT 1000 | The GT 1000 is a cellular-enabled electronic bolt seal that sets the standard for enhanced cargo security and visibility. Codeveloped with the world’s largest seal company, Tyden Brooks, the GT 1000 is targeted for theft prevention and recovery as well as virtual warehouse and in-transit visibility applications for intermodal containers in transit around the world. |
GT 1100 | The award-winning GT 1100 is a self-powered, solar recharging GPS trailer tracking and monitoring solution with low power consumption, long service life in the field and efficient messaging via cellular communications. Its small form factor and flexible design make it easy to install on trailers or in the groove of intermodal containers to enable greater asset visibility and utilization. |
GT 2300 | The GT 2300 is a container tracking device with cellular communications and GPS, providing visibility into the status, movement, loading, and unloading transactions of dry intermodal container operations. By leveraging powerful telematics capabilities integrated with intermodal information systems, domestic container operators can optimize movement and accelerate utilization. |
RT 6000+ | RT 6000+ is a two-way reefer monitoring and control device that provides comprehensive temperature, fuel management, maintenance, and logistical applications services for temperature-controlled cargo. The solution provides visibility, control, and decision rules to transportation companies worldwide, providing maximum compliance, efficiency, and return on investment. |
GT 2000 | The GT 2000 is an economical dual-mode cargo security and monitoring device that enables location tracking and intrusion detection of cargo containers and other assets. The device’s low-profile enclosure facilitates mounting beneath container or trailer locking bars. Security cables can also be threaded through the holes in the metal plate and around the locking bars for added security. |
GT 3000 | The GT 3000 is a dual-mode cargo tracking, security, and situational monitoring device that facilitates near-real-time asset management throughout the supply chain. The device’s highly reliable sensor suite detects intrusion and tampering for maximum container security and visibility. |
GT 3100 | The GT 3100 is a fuel sensor and volume monitoring solution that provides a significant deterrent against fuel theft and pilferage. By providing valuable operations data and reporting from fuel sensors mounted in the tanker, the device enables continuous and reliable monitoring of fuel levels to maximize the security and accountability of fuel transport even in the most remote and hostile areas of the world. |
HE 4000 | The HE 4000 is a ruggedized, dual-mode tracking, and monitoring solution that can used for a broad range of heavy equipment types and sizes in the construction, rail, and utility industries. The device provides accurate and timely status and position information along with key operational metrics, so OEMs, dealers, and end-users can proactively manage their fleet anywhere in the world. |
Globalstar. Globalstar is a provider of mobile satellite voice and data services. Globalstar offers these services to commercial and recreational users in more than 120 countries around the world. The firm’s products include mobile and fixed satellite telephones, simplex and duplex satellite data modems, and flexible service packages. Many land-based and maritime industries benefit from Globalstar services available in remote areas beyond cellular and landline service (Figure 19).
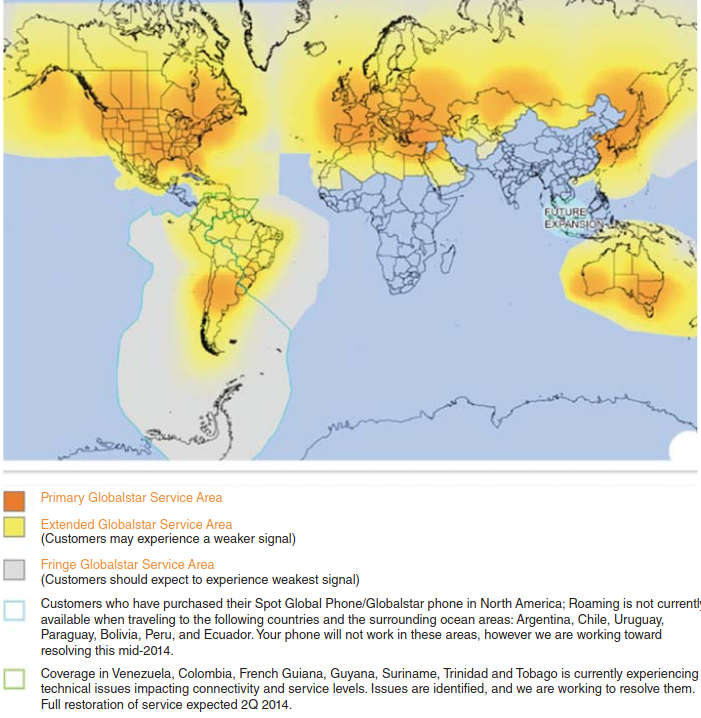
Global customer segments include oil and gas, government, mining, forestry, commercial fishing, utilities, military, transportation, heavy construction, emergency preparedness, and business continuity as well as individual recreational users. Globalstar data solutions are ideal for various asset and personal tracking, data monitoring, and SCADA applications.
Globalstar successfully launched its final batch of second-generation satellites in early 2013. Once placed in service, Globalstar will be positioned as the first MSSs provider to complete the deployment of a second-generation constellation of LEO satellites. Combined with the company’s affordable and award-winning suite of consumer retail SPOT products (e. g., see Figure 20), Globalstar will be uniquely positioned to offer the world’s most extensive lineup of highly reliable and lowest priced MSSs to the broadest range of customers around the globe.
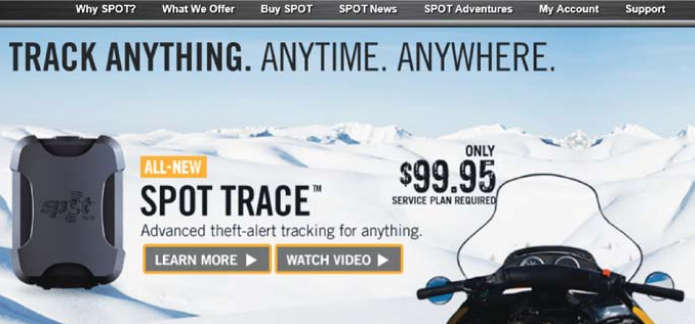
SkyWave. In contrast to Iridium and Globalstar, SkyWave reportedly sees considerable opportunity for ISatDataPro in SCADA applications, and now has a large share of its subscribers using dual-mode satellite-cellular devices. In particular, the SCADA segment has a rather higher ARPU (and is less price sensitive) than SkyWave’s existing ISatM2M subscriber base, especially those using SkyWave solutions as an antitheft device in cars, so even if equipment volumes are slightly lower, SkyWave anticipates substantial revenue growth based on its close relationship with key systems integrators.
Given that a key differentiator between existing BGAN M2M and ISatDataPro terminals, even in the fixed asset monitoring market, is that ISatDataPro operates with a much smaller omnidirectional antenna, if omni-BGAN can come close to ISatDataPro on equipment pricing, while maintaining current per Mbyte price levels ($5–$20 per Mbyte, compared to several hundred dollars per Mbyte for ISatDataPro), then omni-BGAN may end up being in a strong position to compete for SkyWave customers.
SkyBitz. SkyBitz provides global remote asset management solutions, providing real-time information on the location and status of assets. More than 700 enterprises rely on SkyBitz technology to achieve total asset visibility, improved security, lower operating and capital expenses, and enhanced customer service. SkyBitz delivers its solution via SkyBitz Insight, a secure web-based application that is fully customizable and requires no software downloads. SkyBitz specializes in real-time decision-making tools for companies with unpowered assets such as tractor-trailers, intermodal containers, rail cars, power generators, heavy equipment, and other assets. SkyBitz’s asset tracking solution is delivered to commercial, transportation, military, and public safety customers, including sensitive shipment haulers of Arms, Ammunition and Explosives (AA&E) cargos.
As illustrative examples, the SkyBitz Galaxy series GTP1000 and GTP1100 are state-of-the-art, two-way global tracking and monitoring solutions built on the SkyBitz GLS9602 modem platform. The SkyBitz GLS9602 modem combines SkyBitz patented Global Locating System (GLS) technology with the Iridium® 9602 SBD transceiver and can be integrated into a wide variety of asset tracking solutions. These can then provide connectivity to even the most remote locations on earth via the global satellite communications network operated by Iridium Communications Inc. The new SkyBitz Galaxy series delivers operations manager’s total asset and cargo visibility everywhere in the world – even if those assets are unpowered. The solution delivers the visibility required to optimize operations within transportation, oil and gas, chemical, intermodal, government, and other markets that rely on heavy equipment or remote assets.
Founded in 1992 and commercially operated since 2002, SkyBitz has gained valuable operational experience with over 800 large public, private, and public safety customers and hundreds of thousands of assets tracked on a daily basis. SkyBitz leveraged funding from DARPA (Defense Advanced Research Projects Agency) to develop its GLS system and an efficient satellite communications protocol. This protocol provides a more secure platform than traditional GPS and satellite communications solutions. The result is a product that excels in power efficiency, accuracy, and reliability. To save battery power and manage resources more effectively, the SkyBitz GLS-based communicator provides the position of an asset through the computing power of the SkyBitz Data Center, rather than in the device.
ARINC. ARINC This information is based on vendor’s materials.x (now a division of Rockwell) is a provider of aviation connectivity services including flight planning and high-speed data. Their services are captured under the GLOBALink banner of voice and data communications. ARINC developed the Aircraft Communications Addressing and Reporting System (ACARS)™ designed to transmit maintenance data back to the ground. Aeronautical communication typically spans the following elements, among others:
- Ku/Ka satellite connectivity;
- Iridium-based L-band solutions;
- ACARS®;
- Expanded capabilities of VDLM2.
While the services suite encompasses voice and general data transmission, we cover these ARINC services under an M2M rubric because many of the telemetry functions are intrinsically M2M functions, and will likely evolve to M2M-oriented solutions in the future.
The ARINC GLOBALink networks include the VHF ACARS, the VHF Data Link (VDL) system, the High Frequency Data Link (HFDL) system, the Aeronautical Satellite Communications (SATCOM) network, the Iridium Satellite, and GateFusion, an ARINC implementation of the GateLink system characterized in the AEEC standards. VHF data link extends throughout all of North and Central America as well as most of Europe and Asia and is 10 times faster than standard ACARS. Inmarsat satellite service expands VHF capabilities to include real-time reporting of flight and weather information. HF covers remote polar regions, while Iridium is used to fill in intermittent oceanic and polar coverage. Each of these communication subnetworks includes a series of RF channels, multiple ground stations, and a central processor as shared resources for all airline data link avionics.
ACARS is a trusted, proven, reliable communications system used throughout the aviation world. Over 300 airlines and 15 000 aircraft around the world rely on ACARS and the GLOBALink SM infrastructure for these critical communications. The technology has been in use for over 30 years. The system delivers more than 7,5 billion messages a year worldwide; it provides global coverage including North America, Central America, most of Europe and Asia, oceanic, polar, and remote regions.
VDLM2 expands on ACARS capabilities to offer even greater bandwidth and a more extensive suite of flight information, operational control, and ATC applications than ever before. With a capacity more than 10 times that of ACARS, pilots can use the weather graphics, electronic charts, and aircraft monitoring programs now readily available to boost flight efficiency and safety.
ARINC Direct data link communications capabilities include the following:
- Support for all avionics types;
- Uplink flight plans and weather to the FMS;
- Uplink flight plans from ARINC and third-party providers;
- Uplink Coded Departure Routes (CDRs) as needed;
- Send and receive emails, faxes, and text (SMS) messages;
- Message via Type B (IATA) and ICAO (AFTN) networks;
- Concierge and message delivery services;
- Text and graphical weather;
- METARs/TAFs/PIREPs/SIGMETs/NOTAMs, etc.;
- Uplink winds and temperature aloft;
- Aircraft-to-aircraft messaging;
- Email to aircraft.
ARINC Direct SATCOM communications allow operators of private aircraft continuous coverage anywhere. It also provides real-time information on departures, destinations, movement times, engine parameters, delays, positioning, maintenance, and winds aloft.
ARINC’s satellite communications solutions include the following:
- SATCOM voice and data services;
- Inmarsat solutions including support for Aero-H/H+/I, Mini-M, Swift64;
- Solutions for legacy networks such as Aero-C;
- Iridium data link solutions;
- Satellite connectivity to FSS and oceanic ATC facilities;
- Satellite graphical weather support;
- Single-number fleet dialing through ARINC Direct DialTM;
- Automatic detection of incoming faxes;
- Online interface to view real-time activity;
- Seamless L-band and Ku-band switching (vice versa);
- Managed service with no minimum contracts;
- Online interface to view real-time activity;
- Simplified pricing and billing.
As part of a total communications solution, ARINC Direct delivers its customers global data link services using Iridium Data Link Solutions, thus ensuring the timely, accurate delivery of aircraft operational control (AOC) messages. Two-way text messaging, flight movement data, text and graphical weather, Notice to Airmen (NOTAM) alerts, and in-flight route planning are just a few of the applications made possible by Iridium satellite services around the world. Iridium also provides redundancy for satellite services while requiring minimal equipage or upgrade costs, creating a cost effective and vital communications service for your aircraft.
Pilots need to be able to access critical operational and safety information as quickly and efficiently as possible. ARINC offers ATS Data Link Services that makes this possible. ARINC’s ATS solutions minimize cockpit workload while providing accurate real-time information.
Data Link ATS services include the following:
- Predeparture Clearances (PDC and DCL);
- Oceanic Clearance Delivery (OCD);
- FANS, ADS, and CPDLC capability (approved avionics);
- D-ATIS, TWIP, flow reports;
- North Atlantic Track (NAT) messages;
ARINC Direct also supports flight deck weather graphics for a variety of avionics types, including next-generation flight decks with Honeywell Primus Epic® or Rockwell Collins Pro Line 21® avionics suites.
Competitive Wireless Technologies
This section surveys basic wireless technologies to support IoT/M2M applications, as it appears that many such implementations will entail wireless connectivity (rather than wireline connectivity) at the PHY/MAC layer.
Available wireless networks Some refer to the entire “wireless networks” field as Wireless Information and Communication Technology (WICT).x that can be utilized for IoT/M2M applications include the ones listed in Table 6.
Table 6. Key Wireless Technology and Concepts Supporting IoT/M2M Applications | |
---|---|
Technology/Concept | Description |
3GPP (3rd generation partnership project) | 3GPP unites six telecommunications standards bodies, known as “Organizational Partners” and provides their members with a stable environment to produce the highly Reports and Specifications that define 3GPP technologies. These technologies are constantly evolving through – what have become known as – Generations of commercial cellular/mobile systems. 3GPP was originally the standards partnership evolving Global System for Mobile Communication (GSM TM) systems toward the 3rd Generation. However, since the completion of the first Long Term Evolution (LTE) and the Evolved Packet Core specifications, 3GPP has become the focal point for mobile systems beyond 3G. From 3GPP Release 10 onwards – 3GPP is compliant with the latest ITU-R requirements for Internation Mobile Telecommunications (IMT)-Advanced “Systems beyond 3G.” The standard now allows for operation at speeds up to 100 Mbps for high mobility and 1 Gbps for low mobility communication. The original scope of 3GPP was to produce Technical Specifications and Technical Reports for a 3G Mobile System based on evolved GSM core networks and the radio access technologies that they support (i. e., Universal Terrestrial Radio Access (UTRA) both Frequency Division Duplex (FDD) and Time Division Duplex (TDD) modes). The scope was subsequently amended to include the maintenance and development of the GSM TM Technical Specifications and Technical Reports including evolved radio access technologies (e. g., General Packet Radio Service (GPRS) and Enhanced Data rates for GSM Evolution (EDGE)). The term “3GPP specification” covers all GSM (including GPRS and EDGE), Wideband Code Division Multiple Access (W-CDMA), and LTE (including LTE-Advanced) specifications. The following terms are also used to describe networks using the 3G specifications: UMTS Terrestrial Radio Access Network (UTRAN), Universal Mobile Telecommunications System (UMTS) (in Europe), and FOMA (in Japan). |
3GPP2 (third-generation partnership project 2) | 3GPP2 is a collaborative third-generation (3G) telecommunications specifications-setting project comprising North American and Asian interests developing global specifications for ANSI/TIA/EIA-41 Cellular Radiotelecommunication Intersystem Operations network evolution to 3G and global specifications for the radio transmission technologies (RTTs) supported by ANSI/TIA/EIA-41. 3GPP2 was born out of the International Telecommunication Union’s (ITU) “IMT-2000” initiative, covering high speed, broadband, and Internet Protocol (IP)-based mobile systems featuring network-to-network interconnection, feature/service transparency, global roaming, and seamless services independent of location. |
ANT/ANT+ | ANT™ is a low-power proprietary wireless technology introduced in 2004 by the sensor company Dynastream. The system operates in the 2,4 GHz band. ANT devices can operate for years on a coin cell. ANT’s goal is to allow sports and fitness sensors to communicate with a display unit. ANT+™ extends the ANT protocol and makes the devices interoperable in a managed network. ANT+ recently introduced a new certification process as a prerequisite for using ANT+ branding. |
Bluetooth | Bluetooth is a Personal Area Network (PAN) technology based on IEEE 802.15.1. It is a specification for short-range wireless connectivity for portable personal devices initially developed by Ericsson. The Bluetooth Special Interest Group (SIG) made their specifications publicly available in the late 1990s at which time the IEEE 802.15 Group took the Bluetooth work and developed a vendor-independent standard. The sublayers of IEEE 802.15: (i) RF layer; (ii) Baseband layer; (iii) the Link manager; and (iv) the Logical Link Control and Adaptation Protocol (L2CAP). Bluetooth has evolved through four versions; all versions of the Bluetooth standards maintain downward compatibility. Bluetooth Low Energy (BLE) is a subset to Bluetooth v4.0 with an entirely new protocol stack for rapid buildup of simple links. BLE is an alternative to the “power management” features that were introduced in Bluetooth v1.0–v3.0 as part of the standard Bluetooth protocols. (Bluetooth is a trademark of the Bluetooth Alliance, a commercial organization that certifies the interoperability of specific devices designed to the respective IEEE standard.) |
DASH7 | A long-range low-power wireless networking technology, with the following features: • Range: dynamically adjustable from 10 m to 10 km; • Power: <1 mW power draw; • Data Rate: dynamically adjustable from 28 to 200 kbps; • Frequency: 433,92 MHz (available worldwide); • Signal Propagation: penetrates walls, concrete, water; • Real-time locating precision: within 4 m; • Latency: configurable, but worst case is less than 2 s; • P2P messaging; • IPv6 support; • Security: 128-bit AES, public key; • Standard: ISO/IEC 18000-7; advanced by the DASH7 Alliance. |
EDGE (enhanced data rates for global evolution) | An enhancement of the GSM TM radio access technology to provide faster bit rates for data applications, both circuit switched and packet switched. As an enhancement of the existing GSM physical layer, EDGE is realized via modifications of the existing Layer 1 specifications rather than by separate, stand-alone specifications. Other than providing improved data rates, EDGE is transparent to the service offering at the upper layers, but is an enabler for High-Speed Circuit-Switched Data (HSCSD) and Enhanced GPRS (EGPRS). By way of illustration, the General Packet Radio Service (GPRS) can offer a data rate of 115 kbps, whereas EDGE can increase this to 384 kbps. This is comparable with the rate for early implementations of W-CDMA, leading some parties to consider EDGE as a 3G technology rather than 2G (a capability of 384 kbps allows EDGE systems to meet the ITU’s IMT-2000 requirements). EDGE is generally viewed as a bridge between the two generations: a sort of 2,5G. |
General packet radio service (GPRS) | Packet-switched functionality for GSM, which is essentially circuit switched. GPRS is the essential enabler for always-on data connection for applications such as web browsing and Push-to-Talk over Cellular. GPRS was introduced into the GSM specifications in Release 97, and usability was further approved in Releases 98 and 99. It offers faster data rates than plain GSM by aggregating several GSM time slots into a single bearer, potentially up to eight, giving a theoretical data rate of 171 kbps. Most operators do not offer such high rates, because obviously if a slot is being used for a GPRS bearer, it is not available for other traffic. Also, not all mobiles are able to aggregate all combinations of slots. The “GPRS Class Number” indicates the maximum speed capability of a terminal, which might be typically 14 kbps in the uplink direction and 40 kbps in the downlink, comparable with the rates offered by current wireline dial-up modems. Mobile terminals are further classified according to whether or not they can handle simultaneous GSM and GPRS connections: class A = both simultaneously, class B = GPRS connection interrupted during a GSM call, automatically resumed at end of call, class C = manual GSM/GPRS mode switching. Further data rate increases have been achieved with the introduction of EDGE. |
GSM EDGE radio access network (GERAN) | GSM EDGE Radio Access Network (GERAN) is a radio access network architecture based on GSM/EDGE radio access technologies. GERAN is the term given to the second-generation digital cellular GSM radio access technology, including its evolutions in the form of EDGE and, for most purposes, GPRS. The GERAN is harmonized with the UTRAN through a common connectivity to the UMTS core network, making it possible to build a combined network for GSM/GPRS and UMTS. GERAN is also the name of the 3GPP™ Technical Specification Group responsible for its development. Technical Specifications that together comprise a 3GPP system and a GSM/EDGE radio access network are listed in 3GPP TS 41.101. |
IEEE 802.15.4 | IEEE Standard for Local and metropolitan area networks – Part 15.4: Low-Rate Wireless Personal Area Networks (LR-WPANs). IEEE 802.15.4-conformant devices support a wide range of industrial and commercial applications. The amended MAC sublayer facilitates industrial applications such as Process Control and Factory Automation in addition to the MAC behaviors that support the Chinese Wireless Personal Area Network (CWPAN) standard. |
IEEE 802.15.4j (TG4j) medical body area networks | The purpose of Task Group 4j (TG4j) is to create an amendment to 802.15.4, which defines a physical layer for IEEE 802.15.4 in the 2 360–2 400 MHz band and complies with Federal Communications Commission (FCC) MBAN rules. The amendment may also define modifications to the MAC needed to support this new physical layer. This amendment allows 802.15.4- and MAC-defined changes to be used in the MBAN band. |
Infrared data association (IrDA®) | IrDA is a Special Interest Group (SIG) consisting of about 40 members at press time. The SIG is pursuing a 1 Gbps connectivity link; however, this link only operates over a distance of less than 10 cm. One of the challenges with infrared (IR) signaling is its requirement for line-of-sight requirement. In addition, IrDA is also not very power efficient (power per bit) when compared with radio technologies. |
ISA100.11a | ISA SP100 standard for wireless industrial networks developed by the International Society of Automation (ISA) to address all aspects of wireless technologies in a plant. The ISA100 Committee addresses wireless manufacturing and control systems in the areas of the: (i) environment in which the wireless technology is deployed; (ii) technology and life cycle for wireless equipment and systems; (iii) application of Wireless technology. The wireless environment includes the definition of wireless, radio frequencies (starting point), vibration, temperature, humidity, EMC, interoperability, coexistence with existing systems, and physical equipment location. ISA100.11a Working Group Charter addresses: • Low energy consumption devices, with the ability to scale to address large installations; • Wireless infrastructure, interfaces to legacy infrastructure and applications, security, and network management requirements in a functionally scalable manner; • Robustness in the presence of interference found in harsh industrial environments and with legacy systems; • Coexistence with other wireless devices anticipated in the industrial work space; • Interoperability of ISA100 devices. |
Long-term evolution (LTE) | LTE is the 3GPP initiative to evolve the Universal Mobile Telecommunications System (UMTS) technology toward a fourth generation (4G). LTE can be viewed as an architecture framework and a set of ancillary mechanisms that aims at providing seamless IP connectivity between User Equipment (UE) and the packet (IPv4, IPv6) data network without any disruption to the end-users’ applications during mobility. In contrast to the circuit-switched model of previous generation cellular systems, LTE has been designed to support only packet-switched services. |
Near-field communication (NFC) | A group of standards for devices such as PDAs, smartphones, and tablets that support the establishment of wireless communication when such devices are in immediate proximity of a few inches. These standards encompass communications protocols and data exchange formats; they are based on existing radio-frequency identification (RFID) standards including ISO/IEC 14443 and FeliCa (a contactless RFID smart card system developed by Sony, for example, utilized in electronic money cards in use in Japan). NFC standards include ISO/IEC 18092 as well as other standards defined by the NFC Forum. NFC standards allow two-way communication between endpoints (earlier generation systems were one-way systems only. Unpowered NFC-based tags can also be read by NFC devices; therefore, this technology can substitute for earlier one-way systems. Applications of NFC include contactless transactions. |
NIKE+ | Nike+® is a proprietary wireless technology developed by Nike and Apple to allow users to monitor their activity levels while exercising. Its power consumption is relatively high, returning only 40 days of battery life from a coin cell. It is a proprietary radio that only works between Nike and Apple devices. Nike+ devices are shipped as a single unit: processor, radio, and sensor. |
Radio frequency for consumer electronics (RF4CE) | RF4CE is based on ZigBee and was standardized in 2009 by four consumer electronics companies: Sony, Philips, Panasonic, and Samsung. Two silicon vendors support RF4CE: Texas Instruments and Freescale Semiconductor, Inc. RF4CE’s intended use is as a device remote control system, for example, for television set-top boxes. The intention is that it overcomes the common problems associated with infrared: interoperability, line-of-sight, and limited enhanced features. |
Satellite systems | As discussed in this textbook, and specifically in this article, satellite communication plays a key role in commercial, TV/media, government, and military communications because of its intrinsic multicast/broadcast capabilities, mobility aspects, global reach, reliability, and ability to quickly support connectivity in open-space and/or hostile environments. |
UMTS terrestrial radio access network (UTRAN) | A collective term for the NodeBs (base stations) and Radio Network Controllers (RNC) that comprise the UMTS radio access network. NodeB is the equivalent to the base transceiver station (BTS) concept used in GSM. The UTRAN allows connectivity between the UE (user equipment) and the core network. |
Universal mobile telecommunications system (UMTS) | UMTS is a third-generation (3G) mobile cellular technology for networks supporting voice and data (IP) based on the GSM standard developed by the 3GPP (3rd-Generation Partnership Project). |
Wi-Fi | Wireless Local Area networks (LANs) based on the IEEE 802.11 family of standards, including 802.11a, 802.11b, 802.11g, and 802.11n. (Wi-Fi is a trademark of the Wi-Fi Alliance, a commercial organization that certifies the interoperability of specific devices designed to the respective IEEE standard.) |
WiMAX | WiMAX is defined as Worldwide Interoperability for Microwave Access by the WiMAX Forum, formed in June 2001 to promote conformance and interoperability of the IEEE 802.16 standard. The WiMAX Forum describes WiMAX as “a standards-based technology enabling the delivery of last mile wireless broadband access as an alternative to cable and DSL.” |
Wireless M-BUS | The Wireless M-BUS standard (EN 13757-4:2005) specifies communications between water, gas, heat, and electric meters and is becoming widely accepted in Europe for smart metering or Advanced Metering Infrastructure (AMI) applications. Wireless M-BUS is targeted to operate in the 868 MHz band (from 868 to 870 MHz); this band enjoys good trade-offs between RF range and antenna size. Typically, chip manufacturers, for example, Texas Instruments, have both single chip (SoC) and two chip solutions for Wireless M-BUS. |
Wireless sensor network (WSN) | A sensor network is an infrastructure comprising sensing (measuring), computing, and communication elements that gives the administrator the ability to instrument, observe, and react to events and phenomena in a specified environment. Typically, the connectivity is by wireless means, hence the term WSN. |
WirelessHART (aka IEC 62591) | WirelessHART is a wireless sensor networking technology based on the Highway Addressable Remote Transducer Protocol (HART). In 2010, WirelessHart was approved by the International Electrotechnical Commission (IEC) as IEC 62591 as a wireless international standard. IEC 62591 entails operation in the 2,4 GHz ISM band using IEEE 802.15.4 standard radios and makes use of a time-synchronized, self-organizing, and self-healing mesh architecture. WirelessHART/IEC 62591 was defined for the requirements of process field device networks. It is a global IEC-approved standard that specifies an interoperable self-organizing mesh technology in which field devices form wireless networks that dynamically mitigate obstacles in the process environment. This architecture creates a cost-effective automation alternative that does not require wiring and other supporting infrastructure. |
ZigBee RF4CE specification | The specialty-use driven ZigBee RF4CE was designed for simple, two-way device-to-device control applications that do not require the full-featured mesh networking capabilities offered by ZigBee 2007. ZigBee RF4CE offers lower memory size requirements, thereby enabling lower cost implementations. The simple device-to-device topology provides easy development and testing, resulting in faster time to market. ZigBee RF4CE provides a multivendor interoperable solution for consumer electronics featuring a simple, robust, and low-cost communication network for two-way wireless connectivity. Through the ZigBee Certified program, the Alliance independently tests platforms implementing this specification, and has a list of ZigBee Compliant Platforms offering support for ZigBee RF4CE. |
ZigBee specification | The core ZigBee specification defines ZigBee’s smart, cost-effective, and energy-efficient mesh network based on IEEE 802.15.4. It is a self-configuring, self-healing system of redundant, low-cost, very low-power nodes that enable ZigBee’s unique flexibility, mobility, and ease of use. ZigBee is available as two feature sets, ZigBee PRO and ZigBee. Both feature sets define how the ZigBee mesh networks operate. ZigBee PRO, the most widely used specification, is optimized for low power consumption and to support large networks with thousands of devices. (ZigBee is a trademark of the ZigBee Alliance, a commercial organization that certifies the interoperability of specific devices designed to the respective IEEE standard). |
Z-Wave | Z-Wave is a wireless ecosystem that aims at supporting connectivity of home electronics and the user via remote control. It uses low-power radio waves that easily travel through walls, floors, and cabinets. Z-Wave control can be added to almost any electronic device in the home, even devices that one would not ordinarily think of as “intelligent,” such as appliances, window shades, thermostats, smoke alarms, security sensors, and home lighting. Z-Wave operates around 900 MHz (the band used by some cordless telephones but avoids interference with Wi-Fi devices). Z-Wave was developed by Zen-Sys, a Danish startup around 2005; the company was later acquired by Sigma Designs. The Z-Wave Alliance was established in 2005; it comprises about 200 industry leaders dedicated to the development and extension of Z-Wave as the key enabling technology for “smart” home and business applications. |
Many of these technologies are PAN technologies and are not directly applicable to a satellite-focused discussion; however, because 3G/4G cellular (UMTS, GPRS, EDGE, and LTE) can be an alternative to satellite in land mobile environments, a brief description of some of these technologies follows.
Developers of IoT/M2M applications that are geographically dispersed over a city, region, or nation may find cellular networks to be the practical connectivity technology in lieu of the development of specialized new wireless networks. In the near future, M2M applications are expected to become important sources of traffic (and revenues) for cellular data networks. For example, energy suppliers routinely utilize SCADA-based systems to enable remote telemetry functions in the power grid. Traditionally, SCADA systems have used wireline networks to link remote power grid elements with a central operations center; however, at this time and increasing number of utilities are turning to public cellular networks to support these functions. Naturally, reliability and security are key considerations; endpoints typically will support VPN built on IPSec mechanisms in addition to other embedded firewall capabilities.
In starting the discussion about terrestrial mobile networks, one should keep in mind that, as implied in Table 2, IoT/M2M traffic has specific characteristics that relate to the priority of the data being communicated, the size of the data, the real-time streaming needs on one end of the requirements spectrum to the extremely high delay tolerance of the data on the other end of the requirements spectrum, and varying degrees of mobility; cellular/mobile networks are characterized by varying capacity, bandwidth, link conditions, and link utilization, and overall network load, that affect their ability to reliably transfer such M2M data.
These details have to be reconciled in order to be able to cost-effectively utilize cellular technologies for a broad set of applications (while some applications may be less sensitive to cost consideration, many more applications will indeed require optimized connectivity cost metrics). Initial 3GPP efforts have focused on the ability to differentiate MTC-type devices, allowing operators to selectively handle such devices in congestion/overload situations. Specifically, low priority indicator has been added to the relevant UE (User Equipment)-to-network procedures; with this, overload and congestion control are done on both CoN and radio access network based on this indicator.
There are different opinions as to which cellular technologies are practical and/or ideal for M2M. Some proponents claim that many developers are concentrating on 4G products. However, the cost of 4G modules is two times more expensive than 3G modules, and three times more expensive than 2G modules; hence, some proponents only recommend a 4G device if it is going to be deployed in an urban setting, and the cost of connectivity was unimportant. Others argue that if a service provider or organization wanted to deploy an inexpensive system with a short lifespan of 1 or 2 years, they could go with 2G; but if a service provider or organization wanted to build a device to have longevity of around 10 years, then they should consider using 3G.
Universal Mobile Telecommunications System (UMTS)
UMTS is a third-generation (3G) mobile cellular technology for networks supporting voice and data (IP) based on the GSM standard developed by the 3GPP (3rd Generation Partnership Project). UMTS is a component of the ITU IMT-2000 standard set and is functionally comparable with the CDMA2000 standard set for networks based on the competing cdmaOne technology. UMTS can carry many traffic types from real-time Circuit Switched to IP-based Packet Switched.
Universal Terrestrial Radio Access Network (UTRAN) is a collective term for the NodeBs (base stations) and Radio Network Controllers (RNCs) that comprise the UMTS radio access network. NodeB is equivalent to the base transceiver station (BTS) concept used in GSM. The UTRAN allows connectivity between the UE and the CoN. As seen in Figure 21, UTRAN contains the base stations, which are called NodeBs, and the RNC; the RNC provides control functionalities for one or more NodeBs.
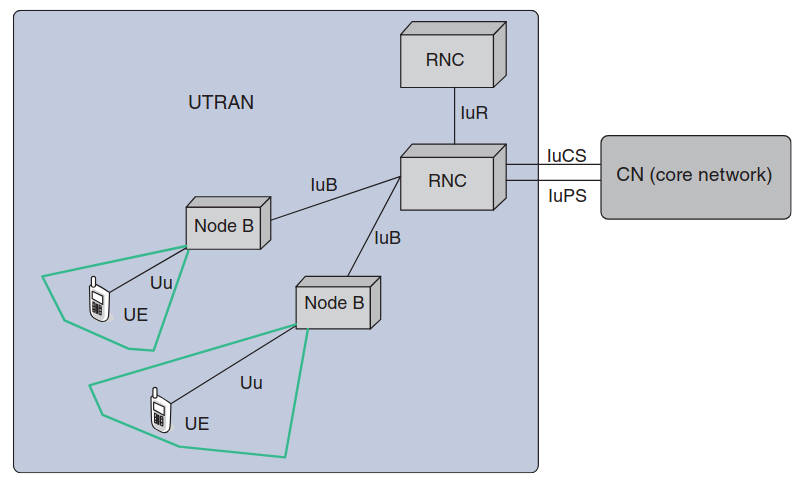
Long-Term Evolution (LTE)
LTE is the 3GPP initiative to evolve the UMTS technology toward a fourth generation (4G). LTE can be viewed as an architecture framework and a set of ancillary mechanisms that aims at providing seamless IP connectivity between UE and the packet (IPv4, IPv6) data network without any disruption to the end-users’ applications during mobility. In contrast to the circuit-switched model of previous generation cellular systems, LTE has been designed to support only packet-switched services.
System Architecture Evolution (SAE) is the corresponding evolution of the GPRS/3G packet CoN evolution. LTE/SAE standards are defined in 3GPP Rel. 8 specifications. Colloquially, the term LTE is typically used to represent both LTE and SAE.
The key element provided by LTE/SAE is the EPS (Evolved Packet System), that is, together LTE and SAE comprise the EPS. EPS provides the user with IP connectivity to a packet data network for accessing the Internet, as well as for supporting services such as streaming video. Figure 22 shows the overall network architecture, including the network elements and the standardized interfaces.
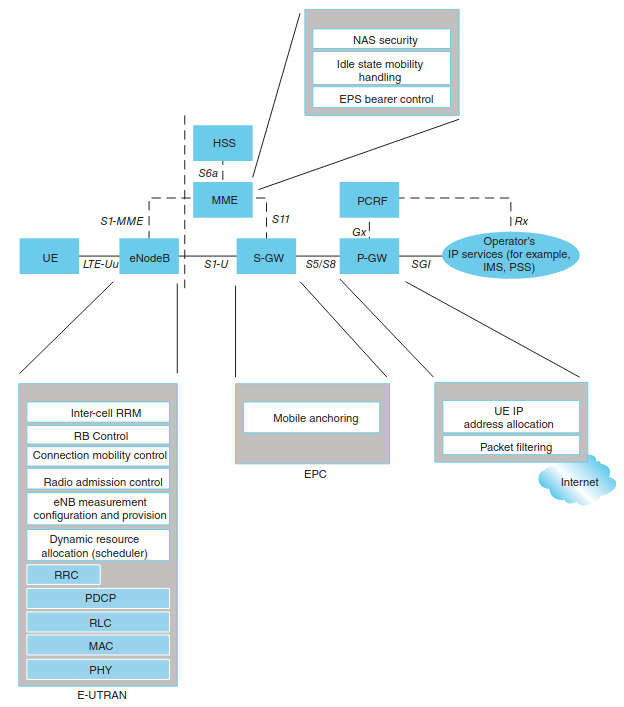
The EPS consists of the following:
- New air interface E-UTRAN (Evolved UTRAN) and;
- The Evolved Packet Core (EPC) network.
Hence, while the term “LTE” encompasses the evolution of the UMTS radio access through the E-UTRAN, it is accompanied by an evolution of the non-radio aspects under the term SAE, which includes, as just noted, the EPC network.
In principle, LTE promises the following benefits:
- Simplified network architecture (Flat IP based);
- Efficient interworking;
- Robust Quality of Service (QoS) framework;
- Common evolution for multiple technologies;
- Real-time, interactive, low-latency true broadband;
- Multisession data;
- End-to-end enhanced QoS management (see below);
- Policy control and management;
- High level of security.
The EPS uses the concept of bearers to route IP traffic from a gateway in the packet data network to the UE. A bearer is an IP packet flow with a defined QoS between the gateway and the UE. The E-UTRAN and EPC together set up and release bearers as required by applications. An EPS bearer is often associated with a QoS. Multiple bearers can be established for an end-user in order to provide different QoS streams or connectivity to different packet data networks or applications reachable via that network. For example, a user might be engaged in watching a video clip while at the same time performing web browsing or FTP download; a video bearer would provide the necessary QoS for the video stream, while a best-effort bearer would be suitable for the web browsing or file transfer session. This is achieved by means of several EPS network elements that have different roles.