Project management is of vital importance to LNG infrastructure projects because of the complex technology, large investments involved, and remote locations of liquefaction plants. There are also needs to source equipment and materials from different countries and coordinate with the supply chain components (e.g., feedgas pipeline, gas liquefaction plant, and marine export terminal). In addition LNG projects typically are developed by multiple contractors and subcontractors and are reliant on suppliers and service companies located around the world, requiring large multicultural labor teams and financial institutions providing large tranches of project finance.
- Introduction
- Project management sequence
- Success of engineering and design
- Sponsor-contractor relationships
- Defining business and project objectives
- The project charter
- Project risk assessments and risk management plans
- Pre-FEED or conceptual risks
- Key project management considerations for LNG projects
- Scenario-based risk assessment
- Technical and extreme risks (engineering and design)
- Hazards and operability (HAZOP) studies
- Project execution risks
- Additional specific precommissioning risk assessments
- Focusing planning stages on constructability
- Developing a project constructability program
- Project cost, schedule estimating, and modeling
- Quality assurance, control, and inspection
- Technology verification and risk-based verification
- Engineering, procurement, and construction (EPC) contracts
- EPC contractor selection and design competitions
- Project management case study: Peru LNG
- Ensuring continuity from project construction to plant operations
- Integrated approaches to achieve high standards of performance
- Conclusions
Introduction
Even with strong project management teams and well-defined project management strategies it is difficult to deliver these projects on time and on budget. This chapter addresses the management of capital projects along the LNG supply chain, focusing particularly on the large-scale facilities associated with liquefaction and regasification plants. For the most part, best practice management for LNG facilities projects follows generic project management principles applicable to most industrial engineering and construction projects. These principles and best practice guidelines are well documented and are addressed here primarily to highlight their importance to the success of LNG projects.
Project management sequence
The sponsors and managers of an LNG facility construction project generally follow a sequence involving a series of defined steps:
- Project definition. Define the plant capacity, feed and product specifications, site location data, and an overview of the conceptual design configurations;
- Project scope. Identify the activities, services, and deliverables that must be provided to fulfill the project definition;
- Project budgeting. Estimate the engineering efforts and costs, including contingencies required to meet the project scope;
- Project planning. Define the engineering milestones that are required to meet the project objectives. The project is staffed with experienced engineers required to produce project deliverables specified in the project scope. Safety, Risks and Security Aspects in Liquefied Natural Gas IndustryProject risks are identified and mitigation plans developed;
- Project scheduling. Estimate the start and completion dates for each activity and sequence of each activity to avoid any bottlenecks. Long lead equipment items are identified for early procurement to meet project schedule;
- Project execution and tracking. Review completeness of the project deliverables, and measure work, time, and costs that have been expended to ensure that the project cost and schedule are on target;
- Project close-out. Plant commission, performance tests, measurement against guarantee values, and final payment per contract terms. The plant is considered successful only when it meets the sponsor’s expectation.
Successful projects require a focused, dedicated, and integrated team led by effective management, which requires clear objectives, a well-thought out plan, realistic stakeholder expectations, effective communication, integrated multicultural workforces, monitoring of project progress, and stakeholder support.
Project management in today’s organizations demands multiskilled persons who can handle and manage far more than their predecessors. They must be capable of resolving project resource problems, interfacing with the clients, as well as making critical decisions in technical areas.
A stages or gates approach to manage a typical LNG project is illustrated in Figure 1. The planning stages (feasibility, pre-FEED, and FEED) must be completed satisfactorily to all stakeholders before the EPC phase that includes detailed engineering, procurement, EPC contracting, construction, fabrication, installation, commissioning, hazards and operability (HAZOP) verification test, and finally, an approval to operate.
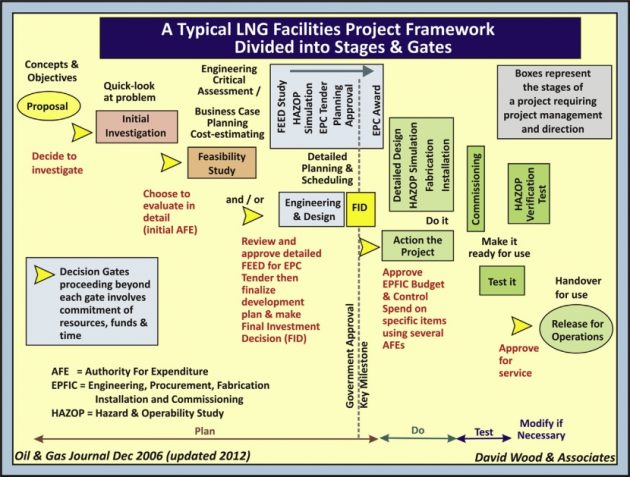
To move from one stage to another requires decisions and approvals to be made on the design selection in respect to the design integrity, plant operability, and environmental impact. Project finance and funding must also be considered. The approval to proceed to a second stage requires AFE (authorities for expenditure) to be signed off by the project owners (and often other stakeholders, e.g., government authorities) and within the project budget. Although the diagram shown in Figure 1 appears to suggest a linear process development from one stage to another, in reality, there are often recycles and redesign of the work completed in earlier stages. However, efforts must be made to minimize the recycles as they may increase the project costs and delay the completion date.
Success of engineering and design
At the onset of a project, the project sponsor team will develop a business case for the project and provide justification to the board of directors for budget approval. Depending on the resources available to the sponsor and the complexity of the project, a consultant may be assigned the task in preparing a recommendation in a study report. Once the recommendation from the study is approved, funding will be released and project definition will be developed. The project sponsor will develop a Design Basis Memorandum (DBM), which typically includes a description of the facility, the feed and product specifications, and the technology selection.
For more complex, large projects that involve developments of infrastructure, acquisition of new technologies, and particularly installation in foreign countries, itis common practice to use consultants to develop a feasibility study and pre-FEED study to further validate the technology selection and plant configuration prior to the FEED phase. These activities must be conducted by competent consulting companies with experienced and knowledgeable staff. It is commonly recognized that the pre-FEED study phase is where most of the cost savings of the project can be realized with minimum man-hours spent.
The technology selection and plant configuration that are defined in the pre-FEED phase will be used as a starting point in the FEED phase. Once the FEED work is started, changes to technology and plant configuration are very costly and may potentially delay the project completion. Changes of the FEED content during the EPC phase are even more difficult, unless the FEED design is proven to be unsafe and inoperable. All these mean that the results from the pre-FEED or FEED phase must be sound, which requires a well-managed team ensuring the success of the project.
Sponsor-contractor relationships
The project sponsor, once satisfied with FEED design, will submit the FEED package to solicit bids from EPC contractors and will select the successful bidder(s). In some cases, project sponsors may enter into cooperation partnerships or joint ventures with an EPC contractor for certain types and sizes of projects. Pricing will be prenegotiated according to a range of possible contract models (fixed price or turnkey, cost-plus, risk-sharing, etc.) or for smaller projects perhaps performed on a reimbursable basis. Both parties, project sponsor and contractor, will set up project teams to do the work. Depending on the finance arrangement, a main EPC contractor, joint venture, or alliance with other EPC subcontractors can be awarded for all aspects of engineering, procurement, and construction or they may be used only to develop detailed engineering and some procurement with the construction carried out by other contractors. Expenditure programs and work package schedules will be set up by the main EPC contractor in consultation with the project sponsor.
The main EPC contractors for LNG facility projects are likely to be internationally known firms like Bechtel, Fluor, Foster Wheeler, KBR, Technip, Worley Parsons, etc. However, smaller contractors can be employed as subcontractors to the project. The main contractor typically has the applicable expertise, experience, and associations with particular technologies (e.g., Bechtel and the ConocoPhillips optimized cascade liquefaction process), but all tend to operate in very similar ways. Consultants and contract personnel are often employed to provide a “cold-eye” review of the design or to support a safety review or engineering studies to supplement the resources or expertise of the main EPC contractor.
Most often, the project sponsors would assign their own staff to the contractor’s home office to monitor the progress and quality of the design efforts.
Read also: Fundamentals of Liquefied Natural Gas
A successful project requires the sponsor and EPC contractor to work closely together. Typically, the sponsor introduces the operation staff at an early stage to ensure that they will accept the design within the confines of the specifications in the design basis and are given the opportunity to review and modify the design as necessary. In this way, the EPC phase can proceed smoothly and would meet the plant operators’ expectations, since they are the ones who will own and run the units. During the construction phase, project management must also address a diverse range of staffing issues, such as union or nonunion staffing, equipment delivery problems, logistic problems, and other unexpected problems that may arise throughout the project.
Most importantly during project execution, the project’s design objectives, cost, schedule, deliverables, and expectations must be clearly communicated to the engineers, employees, sub-contractors, and equipment suppliers.
Defining business and project objectives
The first step in project management is alignment of the business objectives with the project objectives. A project can be installed on time and on budget, but if it does not meet the business objectives, then the project cannot be deemed a success. Some of the questions that the project sponsors should ask include:
- How much gas is available for processing and for how long? Is the quantity sufficient to support the size of an LNG production plant that would satisfy the sales gas purchase agreements? What is the life cycle cost of the installation?;
- What is the projected market value of LNG or gas when the plant is in operation? Has plant downtime been included in the economic analysis?;
- What is a realistic schedule and startup date for the facility?;
- What are the field production pressures, temperatures, and compositions?;
- What are the predicted gas flow rates over the life of the project? Will there be a change in gas pressures, temperatures, and compositions? Will there be an increase in nitrogen content or acid gas content due to enhanced oil recovery upstream?;
- What products can be sold from the facility in addition to LNG/gas (e.g., ethane, propane, butane, condensate, sulphur)? What are the short-term and long-term market value forecasts for these products?;
- What are the specifications of the LNG product? Is there a specification on LNG heating value and/or Wobbe Index?;
- Is there a disposal method that is environmentally acceptable in handling hazardous materials produced from the feedgas, such as mercury, organic sulfur, and other heavy components?;
- How and where will the LNG products be sold (e.g., FOB, CIF, DES contracts)? If products are to be sold on a delivered basis, how will the shipping be conducted (e.g., new-build marine vessels owned/operated by or on behalf of the project sponsor or chartered from existing fleets)? Is the product sold on a BTU basis?;
- Are there any local environmental regulations on emissions and effluents? How do these compare with industry best practice and the project sponsor’s own policies?;
- What are the local safety and security policies requirements? How do these compare with industry best practice and the project sponsor’s own policies?;
- What infrastructure is necessary? This may include roads, bridges, loading and unloading facilities, jetties, port access channels, off-sites and utilities, work force construction camp, permanent operating personnel housing, and recreation facilities;
- Are there any local skilled crafts available for construction, operation, or maintenance?;
- What are the local procurement regulations and mandated requirements for locally hired personnel? Are their skills acceptable to work in LNG project?
Since most LNG facilities form part of a long supply chain stretching across continents with several owners ranging from international oil and gas companies (IOC), national oil and gas companies (NOCs), and utilities companies, the project sponsor may not be able to answer all of these questions, and consultation with the upstream personnel, engineers, permitting agency, government officials, legal entities, and the finance industries is necessary.
The project charter
The project charter is a document often adopted following negotiations between project sponsor and the project management team. It outlines and demonstrates clear project management support for the business and project objectives as outlined by the project sponsor. In particular, it authorizes the project manager or management team to lead the project and allocate resources to contractors/ subcontractors and suppliers as required. The project charter is commonly distributed to all project stakeholders in order to encourage commitment and alignment with the project and its defined objectives. Indeed stakeholder consultations and negotiations may be required prior to the final issue of the project charter in order to ensure alignment with the project as planned. This process should occur early on and may require some compromises on the part of the project sponsor and the project management team in order, for example, to satisfy local community demands. A clearly drafted project charter usually helps with team building and motivation and encourages questions and concerns to be raised and clarifications established early in the project’s schedule. The project charter also has the role of reinforcing the project manager’s and project management team’s authority in conducting the project.
The project sponsor may be a joint venture of organizations (IOCs, NOCs, utilities) with one designated as project operator and in some cases another as technical advisor. The project charter is usually signed off by all joint venture partners forming the project sponsor together with approval of the full project budget and authorities for expenditure (AFE) mandating the project operator to commence the initial stages of the project and the associated expenditures.
Project risk assessments and risk management plans
LNG project developments face many risks that can and frequently do lead to expensive delays. Late delivery by suppliers, contractors, and service providers, prolonged commissioning and plant start-up periods, and other logistical failures can all lead to time and cost overruns that negatively impact a project’s long-term economic value. The complexity of the full LNG supply chaindcomprising upstream facilities, liquefaction plants, a fleet of LNG carriers and, receiving terminalsdmeans that delay or poor performance in any segment of that chain has significant consequences for the performance of the entire supply chain.
A typical 5 MTPA gas liquefaction facility for the whole chain requires a capital expenditure of $7 billion to $10 billion (2 011 dollars), which may incur some $10 million expenditure, but generating product with a value, depending on prevailing prices of natural gas, of some $30 million to $40 million a week. Any delay in delivering the products to the customers represent in a significant loss in cash flow.
Recent analysis has shown that only three of 22 LNG import terminals were delivered on time, while for export terminals, 50% of recent projects experienced significant delays. In addition, reduced performance, commissioning problems, and lengthy startup periods to reach full production have been reported for many gas liquefaction plants in recent years. Delays have ranged typically from 3 to 18 months. The worst performing gas liquefaction projects in this context are, in descending order of delay time with operator/technical leader in brackets: Hammerfest LNG Norway (Statoil), Sakhalin II Russia (Shell), Qatargas 2 (ExxonMobil/QP), Yemen LNG (Total), and Tangguh LNG Indonesia (BP). Note that these projects involve major companies that have had much past experience of delivering large projects. Some projects have been delivered ahead of their planned schedule, such as Egyptian LNG, Equatorial Guinea LNG, and Darwin LNG (Australia), so delay is not inevitable if the risks are well managed. The causes for the delays and cost overruns identified in that study suggested that six categories predominate:
- Political issues and circumstances;
- Design faults;
- LNG deliveries not meeting buyers’ quality specifications;
- Problems with new, unproved technologies;
- Lack of management in addressing issues;
- Unclear interfaces and poor communications between vendors, contractors, and sponsors.
Here we focus on just the following subset of project execution risks associated with specific fa-cilities along the LNG supply chain:
- Pre-FEED or conceptual risks;
- Technical risks (engineering and design);
- Execution risks (construction, installation, startup, plant commissioning, and operations).
However, in practice we recognize that execution risks cannot be considered in isolation from the full spectrum of asset risk and uncertainty.
There are benefits to be gained by identifying execution risks by major project phase and estab-lishing a system that can be used to manage, document, and control risks throughout the project schedule. The aim is to communicate execution risks to management before the start of each major project phase. A definitive process is required in order to systematically and appropriately identify, assess, control, and reduce or mitigate risks. This should also not be confined only to mitigation of downside risks, but also should address the potential exploitation of opportunities (i.e., upside) during all phases of a project.
An integrated Risk Management System (RMS) should address all related project risks and their potential impacts on issues related to a project’s technical quality and performance, cost, schedule, execution, safety, security, health, community, and environment. Risk should be addressed holistically for the project as a whole, including, to the extent that it is possible to predict impacts, all stakeholders, involved parties, all phases, and all types of project exposure and uncertainty. The RMS should be developed in order to properly address all actions to be undertaken to fulfill requirements contained in any and all contractual obligations, and the ongoing incorporation of the results of all assessments and risk mitigation/opportunity exploitation strategies concerned with uncertainties as they become better defined.
Specific equipment operational risks, such as control valve functionality, construction yard loca-tions, or installation sequencing should be captured by HAZOP simulations incorporating detailed risk assessments at the equipment and control level. It is beneficial that such detail be specified in a project’s Risk Management Plan (RMP), which will ultimately be passed on to plant operators once the facility is operational. However, in addition to the technical risk issues covered by the HAZOP, it is prudent for a project RMP to define a Project Execution Plan (PEP), which specifically includes the techniques, processes, plans, and procedures to be used or developed for risk assessment and management during the delivery of a construction project. The PEP should be project specific. Each project should develop a projectwide RMP that defines the specific assessments to be conducted, the timing of the assessments, and the roles and responsibilities of accountable personnel/contractors/suppliers involved. Risk management, though required for all projects, is scalable and each RMP and its component PEP should define the level of detail and analysis that is prudent in the project context.
An Initial Risk Management Plan (IRMP) should be developed to incorporate risk assessments to be conducted prior to detailed project design. This will usually be initiated during a project’s conceptual, feasibility, or pre-FEED analysis. The IRMP should evolve into the RMP, which should be reevaluated and updated on an ongoing basis through to the start of detail design, and again revisited prior to the start of construction/installation activities. A key part of an RMP is that it evolves as a project unfolds through a process incorporating follow-up and/or close-out of all issues identified by the assessments of specific uncertainty events, covering both risks and opportunities.
All companies, small and large, whether they are gas companies, engineering firms, or EPC contractors, should have their own established, comprehensive, and systematic approaches to man-aging project risks. The gas company(s), as the project sponsor (and client of the contractors) and operator, should have its own established system to manage all facets of risks, which needs to be agreed to by its joint venture partners. In addition it should also have detailed coordination procedures that outline the goals and expectations of the contractors’ Risk Management Plans. These coordination procedures should be clearly communicated to the contractors during the EPC tendering phase. Established EPC contractors are expected to have comprehensive risk management programs in place that meet the project sponsor’s requirements and expectations and can be easily integrated with the project sponsor’s RMP.
Pre-FEED or conceptual risks
During the pre-FEED or Conceptual phase an approach should be developed (as part of the IRMP), depending on the project complexity, that seeks to identify a comprehensive list of project issues, possible events, and their likely impacts. The analysis should be systematic, focusing on all aspects of the project, and might best be kicked off by a brainstorming exercise as part of a risk-identification workshop. The scope can be broad and include aspects such as regulatory, fiscal, logistics requirements, geographical locations, technical issues, and so on. This might lead into more detailed hazard identification (HAZID) exercises and some quantitative risk analysis (QRA) of specific issues of concern. See, for example, a discussion of HAZID and QRA results concerning safety drivers associated with FLNG facilities by Shell.
The IRMP should address the resolution of key issues along with guidance on the levels of appropriate senior management involvement. It should also address and document transparently how issues are classified and assimilated. All significant issues (those with substantial negative or positive impacts) need to be brought to the attention of senior management and provisional mitigation strategies and/or contingency plans considered.
Risk assessments during the pre-FEED or Conceptual phase should be performed as set out in the IRMP. As the project progresses, new issues are likely to be identified and analyzed in the same manner. However, as illustrated in Figure 2, the earlier in a project that risk mitigation decisions are taken the more significant their impact is likely to be in controlling ultimate project costs. Late stage decisions focused on risk reduction during detailed design commonly result in cost and time escalations. A comprehensive conceptual risk assessment (CRA) should therefore be initiated prior to a FEED study and long before detailed design.
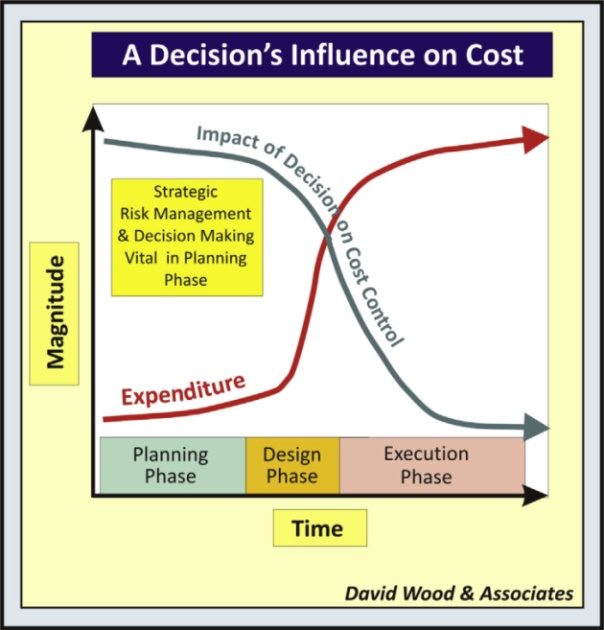
Pre-FEED risk assessment generally involves the appraisal of multiple concepts and multiple cases within each concept. The results of the assessments are utilized to make go or no-go decisions. Additionally, these assessments may simply be a review of a single concept. The purpose is to identify specific areas and further work that needs to be done in the project definition phase and is likely to influence specific equipment and technology selection.
Depending on complexity most LNG facilities projects benefit from a preliminary HAZOP review and simulation. This should be conducted on the facilities design using the design detail available in the draft basis of design (BOD), including layouts, preliminary planned numbered and initialed drawings (PNIDs), equipment data sheets, process flow diagrams, metallurgical flow diagrams, information on likely hazardous materials, and such, as well as the draft project design specifications. The purpose is to identify potential design hazards and special material of construction that need to be addressed in the detailed design phase.
Departures from required asset performances and reliability should be considered during the conceptual risk assessment (CRA). The CRA is based on preliminary drafts of the key early project documents, including the initial project execution plan (IPEP).
This early stage risk analysis enables the project team to move up the learning curve (Figure 3) and reduce the likelihood and potential impacts of identified risk events and in some cases eliminate the causes of potential events.
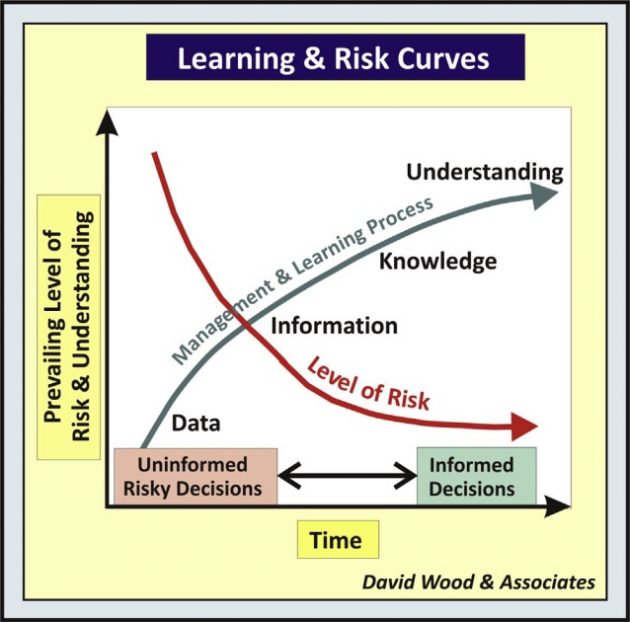
Figures 2 and 3 highlight the conceptual benefits of early stage risk assessment.
Key project management considerations for LNG projects
There are a number of critical issues for sponsors in the design and construction of LNG facilities projects. Some of these issues are technical in nature:
- Selection of technologies;
- Selection of contractors (e.g., lead EPC contractor);
- Development of a project management team;
- Well-considered and communicated strategies for design, contracting, and risk management;
- Cost and budgeting;
- Change orders and deviation to selected designs;
- Scheduling and defining meaningful milestones and targets
- Quality of work and performance.
Other critical issues that impact project performance and outcomes are of a contractual, com-mercial, and financial nature:
- EPC contract structure (e.g., lump sum turnkey or gain share);
- Liability limitations applied to contractors and suppliers;
- Financial security to be posted by contractors (or retained for a period);
- Securing project finance and completion test terms for drawdown of loans;
- Negotiating local content obligations and procurement rules with government;
- Placing the appropriate insurance and export credit guarantees as part of financing.
Scenario-based risk assessment
A scenario-based assessment can be aided by a probability (likelihood) versus consequence (impact) matrix. Such matrices can take several formats varying from purely qualitative to quantitative, with different numbers of individual cells.
Figure 4 shows a 5 x 4 matrix (i.e., 5 alternatives for likelihood of occurrence and 4 alternatives for impact consequences should an event occur). Each cell in the matrix represents a coded outcome with groups of outcomes color-coded, usually using variations of a traffic light approach (red for unacceptably high-risk events requiring mitigation actions and green for acceptably low-risk events requiring little or no control actions).
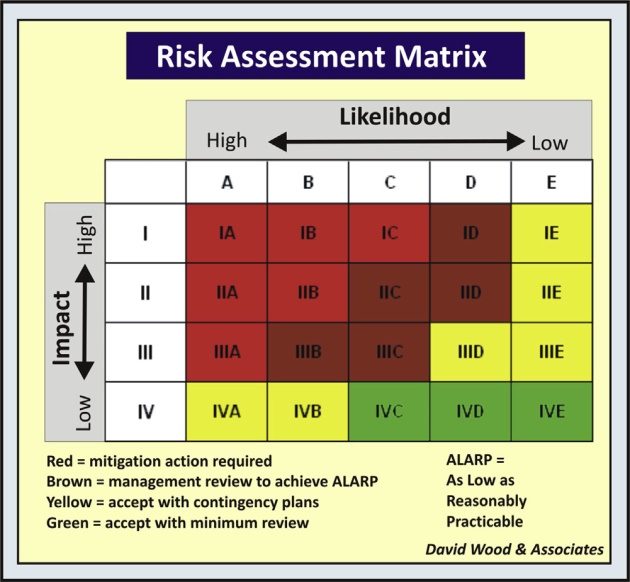
Figures 5 and 6 show example definitions for likelihood and consequence for the matrix illustrated in Figure 4. These definitions and quantitative magnitudes of the impacts are project-specific and it is important that they are defined, understood, and agreed by all involved in project risk assessment.
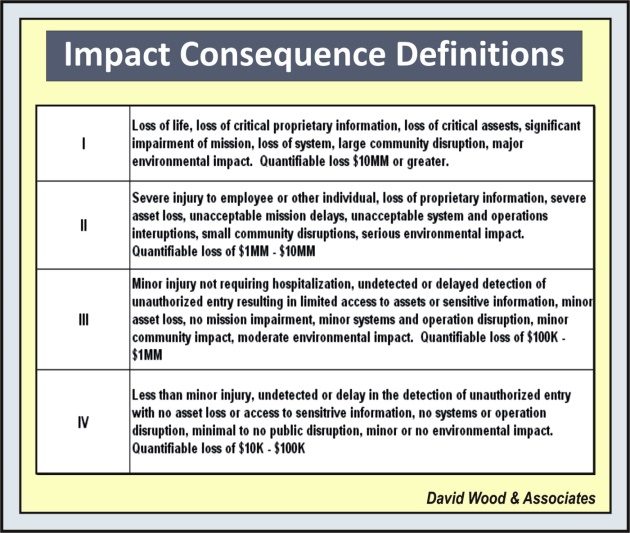
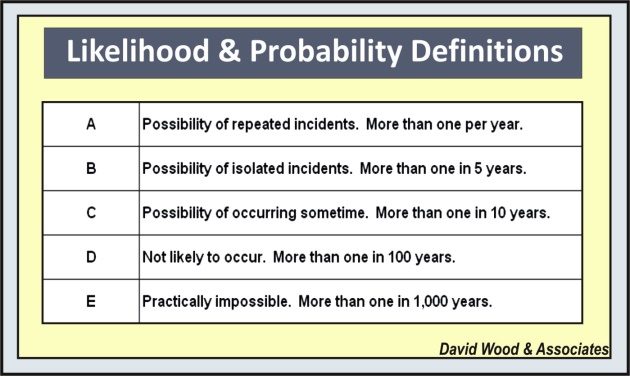
Scenario-based assessments utilizing a risk matrix approach are recommended in order to properly address, rank, and present assessment results for all project phases. It can often be complemented by the use of “bow-tie” and/or “butterfly” diagrams that identify the links between events, their causes and potential consequences/outcomes. Such approaches help in the recognition that events can have multiple outcomes and multiple causes and to focus mitigation strategies on the ultimate causes rather than just the events or their potential outcomes.
Technical and extreme risks (engineering and design)
As a project moves into the definition phase the scenario-based approach can be further developed into an engineering and design risk assessment (EDRA), which should be conducted before initiating the detailed design work. Depending on the size and complexity of the project, the EDRA scope can be very broad and may coverdin addition to specific design aspects such as number of trains, operability, sparing philosophy, and so onda broad range of project aspects such as regulatory approvals and third-party interfaces, contracting strategies, community impacts and relationships, system abandonment at the end of the asset’s operational life, as well as construction. The EDRA may, but does not generally, address specific project details such as control valves or detailed procedures. These are normally covered during HAZOPs and subject to specific risk assessments clearly identified in the RMP. The PEP, work breakdown structure (WBS), BOD, and operating philosophy, all discussed already, are typical project documents that are reviewed and used as the basis of the EDRA.
In the wake of tougher corporate regulation introduced around the world in recent years most large organizations (e.g., gas companies and contractors) have established or expanded risk management procedures and systems integrated with companywide approaches, or enterprise risk management (ERM). The aim of such systems is to make companies more aware of and better prepared to deal with low likelihood but high impact (extreme risk) events should they occur. There is pressure on companies operating in the petroleum industry, from multilateral bodies, governments and some financial institutions to integrating their enterprise risk management frameworks with triple bottom line (3BL) analysis techniques, or the like, to broaden their risk analysis and investment decision processes with more emphasis on corporate social responsibilities (CSR) to improve their performance with respect to communities, staff, environment, health, security, and safety issues. Such developments now require LNG project sponsors and contractors to consider as part of their RMP how they will respond to low likelihood extreme risks and to develop credible contingency and emergency response plans for dealing with such eventualities should they occur. Such credible plans are now more frequently required and reviewed by regulators as part of the project approval and permitting process.
Hazards and operability (HAZOP) studies
HAZOPs are a key ingredient of sound project risk management. HAZOP studies and simulations are normally conducted by the EPC contractors after the design is frozen and/or prior to key deliverables, such as PFDs, PNIDs, and so on, being issued for construction (IFC). However, itis also common for provisional HAZOP studies and/or simulations to be conducted as part of the FEED studies (Figure 1) to confirm that the designs proposed are realistic and operable. All plot plans, layouts, data sheets, and vendor information must be available for review at the start of the HAZOP. The purpose of the HAZOP at the end of the detailed design phase is to surface and address all potential process and operational hazards prior to finalizing equipment and materials procurement and moving into the fabrication, construction, and installation phase. HAZOPs are also conducted to stress test a facility to verify that it performs as per its specified design under a range of possible operating conditions.
A successful HAZOP depends to a large extent on a well-documented, structured process where the complete design is systematically analyzed for deviations from design and operational intent by a team of experienced technical, construction, and operations personnel. The HAZOP team should be ideally independent of the project team or at least impartial when it comes to evaluating design and process performance. The presence and involvement of those individuals to be responsible for operating a facility after commissioning is highly recommended in order to capture all the anticipated operational issues (normal, cold, or hot start ups; normal or emergency shutdowns; normal, temporary, and emergency operations; etc.) and focus them upon responding to potential plant teething problems.
The results of the HAZOP will include a comprehensive list of issues along with recommendations coveringdin addition to engineering and operationsdsafety, health, community, and environmental issues (i.e., in line with ERM and 3BL principles and regulatory standards as applicable; e.g., establishing acceptable quantities of gas flared and other greenhouse gas emissions as part of normal operations).
Responsibility for follow-up/close-out of HAZOP items (coordination of the “list” as well as for each individual item) is documented, understood, and followed up by the project management team to ensure issues are resolved satisfactorily and closed-out in a timely fashion. The HAZOP coordinator/ team leader should agree with actions to be taken and follow up after a specified period of time to ensure the HAZOP recommendations have been addressed.
After completion of the detailed design phase HAZOP, it is a prudent policy to mandate that no changes can be made in engineering, design, construction, or operation that materially affects the results of the HAZOP without a thorough reanalysis. This should be rigorously covered under the project management of change plan (MOCP), including appropriate approvals for initiation, evaluation, and acceptance of changes. All changes introduced after this stage are likely to have significant impacts on project cost and schedule, so ideally should be avoided unless absolutely essential.
All issues and action items stemming from the detailed design phase HAZOP must be ultimately resolved and all impacted project deliverables should be upgraded before the completion of detail design. For the precommissioning HAZOP verification test (Figure 1) all issues and action items stemming from that final HAZOP must be ultimately resolved and all impacted project deliverables should be upgraded before the facility is signed off for operations.
Project execution risks
The Construction Risk Assessment (CRA) is conducted prior to the start of facility construction. The CRA scope can be very broad and may cover all aspects of the construction phase of the project such as government/regulator approvals and interfaces, contractor/subcontractor performance, component integration, weather window implications, interfaces, and issues associated with the final construction plan. The CRA does not usually address very specific project details such as individual yard safety programs, welder qualification, or detailed contractor procedures. These detailed issues are covered by specific risk assessments as identified in the RMP.
Detailed design documents and drawings, construction contracts, supplier quotations, cost estimates, long-lead item schedules, and contractor plans are example input documents that contribute to the CRA. The purpose of the CRA is to identify project level issues and hazards and plans to mitigate the risks and/ or eliminate the causes of events that could have material projectwide impacts. The CRA also often develops into a valuable communication tool, in conjunction with its input to the project risk register, for identifying and documenting project risks to management before starting project construction.
Additional specific precommissioning risk assessments
Other areas of the project subject to specific risk assessments are usually defined in the PEP and RMP. These areas may include, but are not limited to, special design details, contracting strategies, fabrication and load-out activities, heavy lifts, installation activities, early construction work, hook-up and commissioning activities, precommissioning HAZOP, and so on.
All projects suffer exposure to vulnerabilities and uncertaintiesdthese are often encompassed by a project’s or company’s definition of risk. Properly managing this risk exposure requires a structured systematic and holistic approach involving open communication, transparency, accountability from experienced personnel, and flexibility to adjust responses to risks and opportunities as they become identified and more clearly understood.
One of the keys to success is the early identification, assessment, evaluation, and resolution of risk in all phases and at all levels of an LNG project. A solid, tried, and tested method for achieving successful outcomes to project execution is to use the scenario-based approach. In all phases of a project, regardless of the source or which party has liability, there must be a concerted and coordinated effort to work together to manage the risk and share information related to it. If project risks, particularly project execution risks, are not managed through comprehensive assessment and measured responses the consequences frequently come back to haunt all the project stakeholders and can lead to human and financial catastrophes. The LNG sector has an excellent safety track record over the past five decades because close attention has been paid to project risk management at all stages of project development and operation.
Focusing planning stages on constructability
The Construction Industry Institute (CII) defines constructability as
“the optimum use of construction knowledge and experience in planning, design, procurement, and field operations to achieve overall project objectives.”
This emphasizes the importance of putting construction knowledge to work early in the project development and integrating that knowledge with the conceptual, design, and procurement phases to allow for the most efficient, practical, and hence cost-efficient construction techniques to be used. The CII claim that adopting a construction-focused methodology increases the chance of achieving:
- Reduction in total project costs;
- Accelerated schedule to project completion;
- Enhanced plant maintainability, reliability, and operability;
- Selection of safest designs and construction methods.
Like the term “construction-driven,” constructability is not “construction calling the shots.” It is a recognition that the construction phase of a project can constitute up to 50 % of the overall project costs. The construction stage of any facilities project is where the majority of project risks lie (schedule, cost, weather, impacts of new technology/implementation, labor skills, community disputes, etc.). As such, much thought and effort should be expended early in the project so that the most optimum construction methods, techniques, equipment, and materials are used or specified. The criticality and benefits of an early focus on constructability are highlighted in Figure 7.
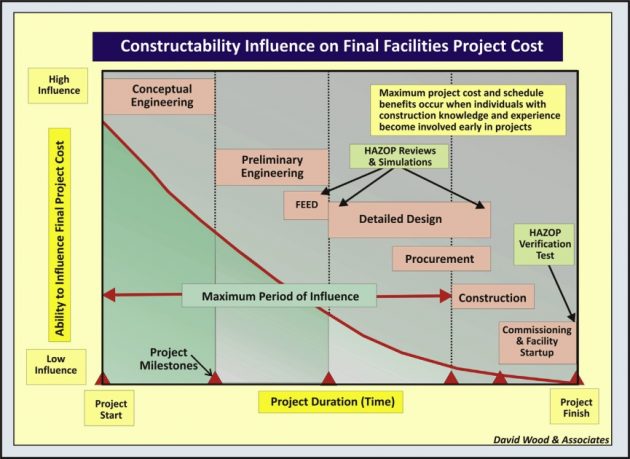
Early stage constructability workshops for routine or repeat projects should include personnel from all participating companies (i.e., operator, joint venture partners, potential construction contractors, subcontractors, service companies, and suppliers) with expertise in construction operations, safety, and appropriate branches of LNG facilities engineering. On larger, more complex, and novel projects the range of skills involved in the workshops would be expanded to include environmental, regulatory, quality, procurement, logistics, health, public relations, legal, and security.
The basic objectives of constructability are to identify, define, and verify:
- Guidelines for engineers, designers, and planners to enhance the probability of a cost-efficient, buildable project;
- Key engineering, design, and procurement deliverables that will have the greatest bearing on the ability to construct the project and to identify where early construction input is needed;
- Construction-related enhancements to engineering, design, and planning that will improve overall safety, quality, costs, and schedule;
- Major execution challenges, uncertainties (risks and opportunities);
- Project contracting strategy and plans for construction/installation considering the specific execution challenges faced;
- Plans for the transfer of constructability information to the next phase of the project.
Regardless of the size of the project or the mix of the participants it is important to have a structured approach, which should be defined in the Constructability Program.
Developing a project constructability program
A constructability program can be developed to outline and document the project management team’s policy regarding constructability. This should consider the minimum criteria required for defining constructability objectives, the general resources roles and responsibilities involved, and a feedback mechanism by which the contribution of the program might be assessed. A constructability program should include at least the following:
- Clearly defined and, where possible, quantified corporate objectives;
- Constructability organization chart defining roles and responsibilities; Constructability plans and implementation guidelines;
- Methodology for key performance indicator (KPI) measurement and verification;
- Analysis of KPIs and interpretation to provide project performance benchmarking;
- Procedures for capturing feedback and learning outcomes;
- Constructability tools:
- orms, agendas, checklists, and workshops;
- Issue and change resolution methodologies;
- Milestones for constructability workshops.
An overview of a typical constructability program timeline is shown in Figure 8.

As project construction kicks off, most of the significant constructability issues should have already been implemented into the construction plans. At this point, a constructability review follow-up action register is often useful to document actions and responsibilities that have been gathered from the formal constructability reviews ongoing through the planning and detailed design phases. A project constructability coordinator should be responsible for working with each manager or supervisor to ensure items are closed-out and properly documented as the project execution plan (PEP) progresses. The project manager or his or her designate should be responsible for ensuring lessons are captured from constructability reviews and discussed with the project constructability coordinator for dissemination to other project teams. In this way the constructability methodology facilitates an ongoing learning and improvement process built into the project strategies incorporated into agreed amendments to the project charter.
Constructability, if properly defined, structured, and used should ensure that construction knowl-edge and experience is used as early as possible in the planning and design process to ensure design and implementation plan practicality and cost effectiveness. Constructability is simply the utilization of construction knowledge, experience, and efficiency in a structured and systematic way throughout the design and execution planning stages of a facilities project.
Constructability issues touch on all aspects of project execution, including safety, scheduling, detailed design, procurement, material/ equipment delivery, contracting, temporary facilities/infrastructure needs, commissioning, and the project management team organization.
Project cost, schedule estimating, and modeling
As project definition improves through the design phases of the project so the uncertainties associated with cost estimates should decrease to a funding level of accuracy of approximately +15 %/-7.5 % with a similar expected range of contingency identified. A probabilistic approach to cost estimating identifying percentiles (P90, P50, and P10) has been used widely used for some time to define cost uncertainties in higher risk projects.
Cost estimating and scheduling software exists to assist with conceptual cost and time estimates as well as detailed estimates and complex project networks involving the optimization of project networks with critical path analysis. Most major EPC contractors have their own custom tools project cost and schedule management tools. Smaller contractors and operating companies may use products supplied by a vendor specializing in these tools. For larger projects it is increasingly common for Monte Carlo simulation analysis to be used in conjunction with project planning software including critical path identification to yield probabilistic estimates of cost and time associated with each project activity and for the project as a whole.
Quality assurance, control, and inspection
An important part in achieving the desired result and performance of a project is the specification and assurance of its quality. The establishment of quality-conscious construction practices is essential with quality control made a priority, ensuring that the required qualities are built into all the tasks. Most established EPC contractors operate integrated Quality, Health, Safety and Environmental (QHSE) management systems, which they apply generically across their operations. This is appropriate for LNG project teams and contractors as it makes clear that all four of its components are important to ensure successful project outcomes and that QHS&E all influence each other as well as the budgetary, schedule, and risk issues that drive project decisions. The adage that “one should expect what one inspects” applies to LNG facilities project management.
Technology verification and risk-based verification
Some facilities deploy new or novel uses of technologies, which can pose significant risks, such as the technology not working as expected based on pilot tests, or requiring modifications that jeopardize the project schedule. Systematic programs to identify, evaluate, and mitigate new-technology risks are available. These systems aim to provide evidence that a new technology being considered will function within specific limits with an acceptable level of confidence. Experience from such technology verification systems suggests:
- Improved end-user confidence in a new technology;
- More efficient use of project resources;
- Systematic identification of failure modes;
- Identification of improvements and design changes at an early stage;
- Optimization of qualification testing and analysis;
- Recognition of interface issues between manufacturer and subvendors;
- Increased likelihood of on-schedule delivery;
- Reduced risk during operation, resulting from fewer uncertainties and increased reliability;
- Traceability of qualification efforts;
- Quicker requalification for new operating conditions.
Risk-based verification schemes provide the ability to focus attention on the high-risk issues that have greatest impact on project cost and schedule and long-term operating performance. Independent verification of projects by competent and qualified engineers and risk managers provides sponsors with the confidence that their projects are designed, constructed, and installed in accordance with acceptable project objectives.
Systems critical to process and safety usually require special attention, particularly during the start-up and initial in-service phase. These systems may require independent verification to ensure integrity and compliance with industry standards. Process systems or equipment delivered from around the world typically need to be verified against project and regulatory requirements before delivery, ensuring unnecessary delays are avoided and items are used safely. Risk-based verification systems facilitate this and thereby aid project management.
Engineering, procurement, and construction (EPC) contracts
The relationship between project sponsors and the contractors they mandate through contractual agreements to engineer, build, and in most cases commission a facility are governed by the negotiated terms of the EPC contracts.
A typical EPC contract contains legal clauses defining the terms and usually a series of attachments and schedules that define the commercial and technical arrangements, such as:
- Scope of work and design basis;
- Conceptual engineering documents;
- FEED study documents;
- Detailed list of contractor deliverables;
- Payment schedule including milestone payments, retentions, and monthly billing process;
- Change order procedures including agreed changes and unilateral changes;
- Subcontractors’ and suppliers’ management and responsibilities;
- Key performance measurement of progress (e.g., earned value);
- Start-up, commissioning, and performance testing;
- Health safety and environment;
- Risk management, including cost escalation, raw materials costs, labor disputes, currency exchange, and interest rates;
- Warranties and performance guarantees;
- Liquidated damages for failure to meet design guarantees;
- Limitations of liability and indemnities (excluding misconduct and gross negligence);
- Insurance;
- Financial security (e.g., parent guarantee, letter of credit, performance bond);
- Dispute resolution and arbitration procedures;
- Project close out procedures.
For an LNG regasification plant for example separate EPC contracts are likely to be required for the following components of the project:
- Site access and basic civil work;
- Soil stabilization;
- Site preparation/civil engineering;
- Constructing LNG storage tanks (main cost of most receiving terminals);
- Marine worksdchannel dredging;
- Unloading jetty and unloading arms;
- Pipework;
- Insulation;
- Buildings;
- Control rooms and instrumentation.
Some issues that are high on the agenda of such projects and require careful attention from the EPC contractors with guidance from the project sponsors are:
- Importance and sensitivity of the project with respect to government and local community;
- Regulatory compliance;
- Safety, security, and environmental standards;
- Health standards for labor force (policies on exposure to extreme conditions);
- Precautions against diseases, pests, and vermin;
- Local staffing regulations and indigenous contractor and supplier priorities;
- Nonmanual staffing strategies and interfaces with sponsor’s project team;
- Site access and supply logistics.
Change management requires particular attention and can be greatly helped by early warnings of potential problems and likely changes. The key performance measurements collected on an on-going basis throughout a project help in this early identification process. This facilitates informed and timely decisions on change orders with the sponsor made fully aware of the cost implications of any unilateral late-stage change orders imposed on the project. The aim is to minimize late stage surprises in order to avoid cost overruns and delays.
It pays to have the customer deliverables of projects clearly spelled out in an EPC contract addressing at least the following:
- What is actually to be delivered (a detailed list of items);
- In what format, specification, and condition is it to be delivered;
- A review process to report and evaluate delivery as it progresses;
- How is delivery to be approved and confirmed as being acceptable to the customer or sponsor;
- How and when project and plant documents are to be handed over to the sponsor or plant operating team.
Relationships between the EPC contractors and project sponsor or customer require specific attention and joint team building initiatives from both contractors and sponsors.
Contractors prefer to have:
- A single point of contact with the sponsor or customer;
- Formal lines of communication and notification regarding deliverables;
- Timely and well-communicated decision-making from the sponsor;
- Alignment within the project sponsor team.
Sponsor and customer expectations of EPC contractors include:
- Timely delivery to the project plan (within budget and on schedule);
- Clear understanding of what the project sponsor wants, not what the contractor would prefer to deliver;
- Understanding of the project’s goals and decisions as specified in the EPC contract;
- Active listening to the project sponsor’s management team and proactively taking on board responsibilities for issues as and when they arise.
Well-drafted EPC contracts greatly aid the integration and alignment of a project team.
EPC contractor selection and design competitions
EPC contractors are typically selected by a competitive bidding process or by bilateral negotiations between the sponsor and a single favored contractor. Procurement regulations in a specific country may specifically require a bidding process with a prequalification exercise to establish whether local companies are able to provide the desired deliverables. If it is through a bid process it is usual to award the FEED study to one company and to have that company excluded from the bid list for the EPC contracts. This avoids conflicts of interest or manipulation in the design specifications.
Competitive bidding takes time and may reduce flexibility in terms of the contractor ultimately being able to deliver the sponsor a tailored product. Nevertheless, competition does tend to drive down prices over time, but that depends on the conditions in the market. If the contractors’ order books contain plenty of work and upcoming projects already awarded, some will elect not to compete in a time-consuming and costly (i.e., for the contractors) process with modest chances of success; others may just bid high on the basis that they will accept the work only if it offers them high margins.
Read also: Overview, Process and Technology of Natural Gas Liquefaction (NLG)
Bilateral, sometimes open-book, negotiations can save time avoiding a bidding schedule, motivate the contractors to provide innovative solutions, and deliver a successful project. They are unlikely to deliver the lowest price budget, but if the sponsor and contractor have long-term relationships, or are trying to develop one, competition is not needed to motivate contractor performance. Contractor reputation counts for a lot regarding whether sponsors are prepared to deal with them on an open-book basis, leading ultimately to a lump-sum price for the contract.
There are in fact several alternative strategies that can be used to select EPC contractors. The most common options include one or more of the following:
- Negotiate a project with a preferred contractor;
- Competitively bid every phase of the project;
- Design competition for FEED followed by lump sum EPC bidding;
- Reimbursable EPC payment terms;
- Owner-contractor alliance-based execution (e.g., gain share contracts).
In bilaterally negotiated contracts the FEED and EPC work is typically done by the same tried and tested contractor. Additional advantages of this approach are that the sponsor does not have to pay bid compensation to multiple contractors and it gives the sponsor’s team more time to focus on other project issues. Also the sponsor participates fully in the development of the EPC work program, cost estimate, schedule, budget, and contingencies. The sponsor should therefore gain a good insight into the uncertainties associated with those key items.
The most commonly used approach for selecting an EPC contractor for a gas liquefaction facility involves the development of a FEED package with one contractor followed by competitive bidding for the EPC contract (see Figure 9). Typically with this approach, a FEED contractor is competitively selected based on a qualitative evaluation, which includes past experience, evaluation of key personnel, price, and execution plans. The scope of deliverables for the FEED study are not usually determined competitively, although the liquefaction process technology may be preselected by the project sponsors with advice from a competent engineer or contractor during the pre-FEED phase. The FEED deliverables are then competitively bid in order to obtain lump sum EPC proposals from prequalified contractors.
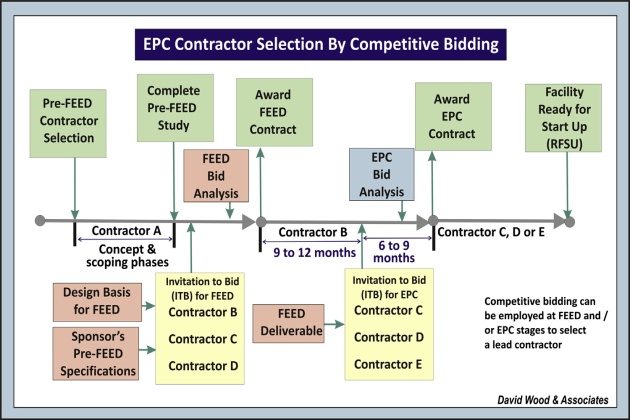
Another approach involves selecting more than one contractor to develop parallel FEED studies. Each FEED contractor commits to generate a lump sum bid for the EPC contract based on their respective FEED package. This process is referred to as a design competition (Figure 10). This strategy was adopted for the SEGAS liquefaction plant built in Egypt. There is no guarantee, however, that a design competition will lead to the lowest $/tonne of capacity, but as the FEED stage of the process is identified as the most important in influencing cost and identifying risks in time for them to be appropriately managed, having more than one FEED study conducted by different contractors has its appeal to project sponsors.
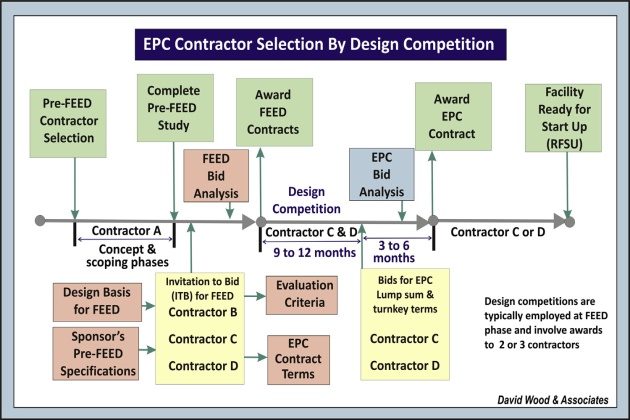
Project sponsors have to set rules and guidelines for the participants in conducting design competitions. To do so the following factors require careful consideration:
- Plant components for which the sponsor is prepared to entertain new or novel designs or configurations should be outlined at a high level in advance of the studies. Where ideas that would be considered new or novel are proposed, the onus should be on the design team to identify what steps would be taken to identify, minimize, or eliminate the associated risks;
- All the designs competing in the design competition should be based on process technologies that have been rigorously tested to at least “prototype” (a defined term in each study) level;
- All FEEDs should be required to use the same design basis to ensure a level playing field among the design teams. The design basis requires careful definition by the project sponsor. This can require quite onerous specification guidelines to be prepared in advance by the sponsor;
- The rules set at the beginning of design competitions should be closely followed to ensure fairness and integrity to the competitive process. Each team should be given the same level of information and guidance from the sponsor;
- The sponsor should define in advance the evaluation criteria prior to the start of the FEED. This allows the contractor teams to understand what is important to the owners and this ensures that the work done during the FEED is consistent with the owner requirements. These evaluation criteria provide the contractors with the basis to optimize the design and risk management;
- Sponsors should determine sparing philosophy, requirements for parallel (2-in-1) train designs that decide plant availability along with a list of acceptable equipment vendors as part of the design competition guidelines. This should result in bids that can be more easily compared;
- The sponsor should define in advance the key legal terms and conditions for the EPC contract as these will have an impact on the contractors’ lump sum bids. For example, high liquidated damages for missing contractually guaranteed plant capacity values tend to increase the margins applied by the contractors when formulating their bids. If some tolerance is granted in achieving guaranteed specifications then contractors tend to reduce the margins added to the design or to project contingency lump sums, promoting a more fit-for-purpose design philosophy;
- Owners should ensure equal treatment for the competing teams in respect of design scope, compensation for the scope, schedule, and terms/conditions for the contract;
- All the competing EPC bids and FEEDs should be submitted at the same time. Sequential bidding and reverse auctions should be avoided. The contractors should be required to submit only one EPC estimate, on the basis that multiple EPC lump-sum estimates from each bidder compromises the competitive process;
- All contractors should be free to choose partners, suppliers, and subcontractors from an approved or prequalified list. Project sponsors should ensure key participants are free to compete in either design to avoid potential advantage or disadvantage of one design team over the other. If there are particular suppliers or subcontractors that have a definite commercial advantage, then they should be forced to work with each competing design team.
Even though design competitions have the potential to be very effective in achieving sponsor’s EPC objectives and goals for the project, they have to be carefully conducted and managed. Trust and fairness ensure that all parties are aligned and work towards the benefit of the project. Structuring a design competition to achieve maximum benefit is not easy and requires the sponsor to balance their desire to specify the design versus encouraging the contractor to apply their knowledge and experience and be innovative.
Project management case study: Peru LNG
The Peru LNG Liquefaction Plant at Pampa Melchorita (Peru) was commissioned in 2009. A detailed case study describing the project and its evolution is provided by Bruce and Lopez-Pinon (2009). The information discussed here is drawn from that detailed study. The project is of interest because it was delivered on budget during a time of rapidly escalating costs, which impacted most other projects executed at the same time.
The key stated project driver for the project management team was cost optimization and this worked in delivering the project at a unit EPC (and commissioning) cost of $420/ ton of annual LNG production capacity (including both gas liquefaction plant and marine terminal). Mayer (2011) of CB&I quoted a $440/ton cost in 2008 dollars. This is at the lower end of a trend that extends up to some $1400/ton for other plants built at the same time elsewhere in the world. The Peru LNG project consists of the liquefaction plant, a marine terminal, and a gas supply pipeline. It was the largest single investment ever in Peru. At $3.8 billion, the total project cost including project finance costs constituted the largest project finance in Latin America, with loans obtained up to $ 2.2 billion.
The project was developed over eight years through a challenging period in which the project had to overcome:
- A steep global capital cost inflation trend;
- Extended delivery times for equipment and materials;
- Shortages of skilled labor and experienced contractors, due to competition from other projects around the world;
- Stringent environmental and social conditions;
- Opposition from some sectors of the community;
- Difficult conditions for securing project financing.
Despite those difficulties PERU LNG project was completed as specified for production capacity, Energy consumption Optimization and Improvements in the Natural Gas Liquefaction Processenergy efficiency, and plant reliability; within the approved budget; and in accordance with the time schedule specified at FID.
Conceptual studies were conducted in 2001, which confirmed the technical feasibility and eco-nomic viability of the project. KBR performed a FEED study for the sponsors in 2002 based upon the proven and popular Air Product’s C3MR gas liquefaction technology. The FEED also confirmed that air cooling and gas turbine drivers were the most efficient and reliable solutions for the Peruvian plant.
A site for the liquefaction plant and marine export terminal was selected at Pampa Melchorita, 170 km south of Lima. In 2003 physical and geotechnical studies on the selected sitedboth onshore and offshoredand the Environmental Impact Assessment (EIA) was initiated and continued through 2004 and 2005 in order to obtain final approval by Peruvian authorities and potential lender agencies. During that period plant design optimization and technology improvement works were made to the FEED specifications.
Read also: Dynamic Simulation and Optimization of LNG Plants and Import Terminals
While the EIA work was conducted PERU LNG negotiated the Commercial framework for the project. Three keenly negotiated agreements were established:
- A feedgas agreement for the supply of 620 MMscfd with the joint venture holding the Camisea gas fields. This involved a gas sales agreement signed in 2005 for gas to be acquired FOB the gas treatment plant at the gas fields some 600 km from the Peru LNG site, with initial supply of gas scheduled for March 2010;
- All LNG produced by the liquefaction plant was sold to Repsoldwith a minimum annual quantity of 4.2 MTPAdthrough a FOB gas sales and purchase agreement (SPA) signed in June 2005, with the first cargo lifting date set for May 2010;
- The legal framework for the project, an agreed stable fiscal regime over the life of the project, investment requirements (local content), and some other supporting provisions, was executed between Peru LNG and the government of Peru, in the form of an Investment Agreement (contract) executed in January 2006.
These three critical agreements were supplemented by two further commercial agreements that bolstered the LNG offtake agreement:
- In September 2007 REPSOL entered an agreement with Mexican state-owned “Comision Federal de Electricidad” (CFE) to supply LNG to the Manzanillo regasification terminal, located on the Mexican Pacific coast. Initial regasification capacity of that terminal is 500 MMscfd. Construction of the Manzanillo terminal started in July 2008 with a completion date scheduled for the end of 2011 with an estimated investment of over $700 million (it was actually delayed by a few months in coming online);
- An agreement for the LNG tankers for this trade to be supplied by STREAM (50 % Repsol, 50 % Gas Natural of Spain) under long-term charters with ship owners. Three newly built dual fuel vessels are dedicated to this project, with an additional vessel available if necessary. Each vessel is capable of loading 173,400 m3 of LNG. Expected delivery date for the first LNG tanker is March 2010.
Peru LNG executed extreme care when selecting and organizing its project management team. The emphasis was on a team of experienced and professional individuals within a simple team organization structure. Classic project management techniques were to be the backbone of the project management philosophy, namely:
- Project planning;
- Task scheduling;
- Cost budgeting;
- Design optimization;
- Contract strategy;
- Risk profile definition;
- Contractor selection.
Prioritizing these items played a fundamental role in the success of the PERU LNG project.
The most challenging difficulty faced by the gas liquefaction plant project was the high seismic activity exposure of the site location. As an example in August 2007, during initial construction works, the Peruvian coast experienced an earthquake registering 7.9 degrees on the Richter scale with its epicenter less than 30 km away from the site. Consequently the plant had to be designed to safely withstand an 8.6 degree earthquake with neither damage nor loss of containment. Specially designed tank foundations (e.g., including a tank base with special seismic isolators), thicker concrete rebar, increased sections of structural steel, and bracing of equipment and valves were some of the measures implemented to deal with the issue.
Other challenges included:
- Numerous geological and terrain hazards along the entire feedgas pipeline route varying from very steep slopes to unstable slopes and landslides, and from moving sand dunes to torrential rivers. This PERU LNG pipeline is one of the highest altitude pipelines ever built reaching an elevation of 4,901 m above sea level. The Peru LNG pipeline has a diameter of 34 in and is 408 km long. It is currently capable of delivering 1.2 billion scf/day at the Pampa Melchorita delivery point, of which approximately 700 MMscfd are dedicated to the LNG production and up to 500 MMscfd are for third-party transportation;
- The environmental impact commitments went far beyond the requirements of existing regulations and compliance with environmental standards in order to secure government and lenders’ approvals. The additional requirements were specified especially on the social/economical side. In addition as work progressed local municipalities and local communities along the pipeline route expected the Peru LNG to fulfill their demands in terms of special taxes, monetary compensations, additional jobs for their people, or social investments. Managing these demands and requests in a way to minimize time delays and additional Liquefied Natural Gas Projects, calculation of the cost of gas productionproject costs required care and diplomacy skills.
The gas liquefaction plant site occupies 520 ha of desert land adjacent to the Peruvian coast 170 km south of Lima, at 135 m average altitude. It lies just off the Pan-American Highway, in between the towns of Canete (30,000 inhabitants) and Chincha (over 100,000 inhabitants). The site is 40 km north of Pisco port where most equipment and materials for the project were unloaded during construction time. Climate is very mild with temperatures never exceeding 28 °C nor dropping below 12 °C, and practically no rain year round, though humidity is always well over 80 %. Besides the highway no other infrastructure was available at the site.
Feed gas supplying the plant is very lean and dry (well above hydrocarbon and water dew points) with C3+ content below mol. 0.02 %. Consequently no NGL fractionation is necessary at the site. The plant is self-sufficient as it generates all the electricity it requires and produces all the water, nitrogen, and compressed air that are needed, as well as taking care of liquid effluents and solid wastes. A permanent community with housing and recreation facilities for all members of the operational staff is part of the project.
The current project consists of the installation of a single liquefaction train, though provisions for expansion to include and tie-in additional trains were part of the design. The LNG loading line slopes down 140 m from the tanks to the beach and then it extends for another 1.3 km up to the loading arms; PERU LNG adopted a special piping design for this line and incorporated quick opening valves and a surge drum at the loading platform.
The marine terminal is designed to handle LNG tankers ranging in capacity from 90,000 up to 175,000 m3. It consists of following components:
- A 1.3 km long jetty into the Pacific Ocean;
- The loading platform and ships dock;
- Four breasting and six mooring dolphins;
- 3.6 km dredged navigation channel 18 m deep;
- 800 m long breakwater protecting the whole harbor;
- Tug boat dock, with a 200 m long breakwater;
- Mooring and navigation aids and ancillary equipment.
The 1.3 km long trestle consists of a two-lane road and a steel rack containing the LNG, with boil- off and other pipes running all the way to the loading platform. Both road and rack are supported by an extra strong steel structure that is anchored to the sea bottom by 550 steel piles that were driven into the soil down to the rock substratum. The main breakwater is located 1,600 m from the shore at more than 15 m of water depth, and stands up to 11 m over the lowest (at spring tide) water level in order to guarantee protection to the docked LNG tankers against the incoming ocean wavesdup to the 100-year return design level.
The design criteria agreed upon was that the liquefaction plant should produce LNG at a rate above contracted sales (not less than 4.2 MTA net of losses) in an efficient manner (i.e., total fuel consumption + losses under 9 %) with a high plant availability (i.e., above 94 %/year) and ensuring that it would achieve that in a safe manner and free of environmental impacts (i.e., tightly controlled emissions and prevention of spills). A financing objective was to involve 40 % equity and secure 60 % debt finance to support the capital investment.
The conditions of the investment agreement with the government included:
- Creation of 12,000 direct jobs plus an estimate of 30,000 indirect in the country, during construction (at peak time);
- Contracts awarded and goods purchased from Peruvian companies for more than $1 billion;
- A net contribution of 0.6 % growth to the nation GDP;
- Job training for thousands of Peruvian nationals in the fields of civil engineering, pipe and structural welding, electrical and instrumentation, etc.
In addition it was estimated that some $7.8 billion in royalties and taxes to the government would be generated by LNG sales throughout the life of the project.
Following EIA approvals and Offshore supply chain of Liquefied Natural Gassupply chain commercial agreements being secured, project implementation commenced in earnest in mid-2005. At that time several activities were launched, namely:
- A project management organization was established and key positions filled;
- Plant site preparation works (Phase I) were awarded;
- Gas liquefaction plant EPC lump sum turnkey bids were requested from KBR (open book);
- Bid packages were prepared for planned early awards as follows: Site preparation (Phase II) to several local bidders, MCHE supply (Air Products), and Refrigeration compressors supply (G.E. and Nuovo Pignone);
- Marine terminal EPC package was prepared for launching a lump sum turnkey bid request (several international bidders);
- A pipeline engineering bid package was prepared and sent to several bidders.
Almost two years were consumed in the bidding process for the gas liquefaction plant Engineering, Procurement, Construction and Commissioning (EPCC) contract, with lengthy negotiations being undertaken with KBR and Chicago Bridge & Iron (CB&I). Finally in December 2006 PERU LNG awarded the work to CB&I on a lump sum basis, with Notice to Proceed issued in January 2007, for a price of $1.6 billion (wholly including MCHE (Air Products) and Refrigeration compressors (Nuovo Pignone) early awards). CB&I then had 40 months EPCC execution time to First LNG Cargo.
Following detailed engineering at its London and Chicago offices, CB&I procured and installed following main equipment and liquefaction plant components:
- Process and pressure vessels:
- 44;
- Heat exchangers (including MCHE):
- 19;
- Air coolers:
- 228;
- Pumps and compressors (with drivers):
- 57;
- Electrical units:
- 295;
- Tanks (including those built on site):
- 16;
- Valves:
- 3,610;
- Instruments:
- 1,870.
- Buildings (process and nonprocess):
- 24, plus the permanent accommodation for the plant operating community. Only four significant cost deviations occurred in the total project (i.e., pipeline, liquefaction plant, and marine terminal) from when it was approved in 2006, namely:
- An increase in marine EPC contract (CDB) price driven mainly by a geotechnical problem that was only evident during construction;
- An increase in pipeline construction contract (Techint) price due to unexpected work stoppages caused by local communities along the rights of way (ROW);
- An increase in staff and third-party services costs as a consequence of additional archaeological and social work in relation to the pipeline project;
- A decrease in financing costs mainly due to lower than predicted interest rates during construction; the savings in financing just offset the preceding three increases in project costs.
The gas liquefaction plant EPC lump sum price contract with CB&I did experience several change variations during project execution but these did not significantly impact the contract value from its award value (i.e., $1.6 billion budget; Dec, 2006). PERU LNG managed to deliver this gas liquefaction plant, capable of producing some 4.5 MTPA, by focusing on fundamental principles of project management (e.g., design optimization, anticipation in decision-making, competition in EPCs, and all other contracts and rigorous planning schedules). It also benefitted from full support and knowledgeable collaboration from all project stakeholders and exceptional performance from Peruvian construction workers.
Some key contributing factors to this successful outcome, as detailed by Bruce and Lopez-Pinon (2009) were:
- Cost reduction associated with a safe, reliable, and simple (1 train) design using proven technology;
- Establishing EPCC competition for the key contracts;
- Planning ahead; for example, timely land acquisition and securing rights of way (pipeline)
- Allocation of time and resources to commercial and contractual negotiations;
- Anticipation of time-consuming processes in the environmental impact studies, meeting corporate social responsibility obligations, and securing project finance agreements;
- Early selection of gas liquefaction plant site;
- Different fit-for-purpose contracting strategies adopted, based on previous experience, for the pipeline, liquefaction plant, and marine terminal components of the project. The initial open-book EPCC contract negotiations with KBR for the liquefaction plant failed to deliver acceptable commercial and contractual terms and were replaced promptly with open-book negotiated agreements with CB&I;
- Careful capital cost benchmarking against other comparable-scale liquefaction projects being specified in the 2003 to 2005 period (e.g., Damietta in Egypt and Skikda and Arzew plants in Algeria) proved vital in establishing a realistic project budget.
Taking into account the risks that contractors were taking in adopting lump sum turnkey contracts for EPC projects in an environment experiencing rapid inflation and rising prices for raw materials in 2005-2006, it is not surprising perhaps that EPC negotiations proved difficult for both sponsor and contractors.
Other published case studies providing significant insight to LNG facilities project management recommended for review are Technip’s account of delivering mega-liquefaction projects in Qatar for Qatar Petroleum and ExxonMobil and the description of the ground-breaking Atlantic LNG train 1 project in Trinidad, deploying the Phillips optimized cascade liquefaction technology for the first time for sponsors BP and partners.
Ensuring continuity from project construction to plant operations
Handing over from the development and construction workforce to a plant’s operational team can be one of the most challenging aspects of managing any project. Capturing and transferring highly-detailed and specific knowledge of a new plant, its machinery, its suppliers, and its commercial contracts can be a complex, subtle process. It is important for ensuring continuity, safety, and long-term plant performance.
Training and retaining operations staff in remote facilities where local skills may be in short supply is not an easy task. This can be complicated by rules that require skilled staff to be primarily drawn from the local and national population. Good terms and conditions, long-term prospects and desirable housing and, potentially, schooling, onsite for employees and their families are required to retain skilled plant operators.
Operations personnel are often embedded into inspection and construction teams leading up to a project’s startup. This ensures that final HAZOP testing, precommissioning, and commissioning are all witnessed, and in some cases, primarily handled by the core of the future operations team.
Operations management systems (OMS) are an essential part of ensuring that plant operations and the operating team are fit-for-purpose and able to operate the plant safely according to clearly specified guidelines.
Addressing the OMS early ensures that the system is ready to use during the critical facility-commissioning phase and helps avoid startup delays and incidents. A draft OMS should be one of the deliverables of the precommissioning activities and further developed by the future operating team. Indeed some projects recognize the need for an OMS and start to plan and design it as early as the FEED stage of a project. Such an approach ensures that the OMS is developed and available when required and provides accurate, real-time information to enhance decision-making.
An operator training simulator (OTS) can also be developed and maintained for training of new plant operators and analyzing plant performance under specific conditions relevant to planned operations. The long-term benefits of investing in an operator training simulator according to KBR are:
- Operator best practices are reinforced through refresher training;
- Workforce flexibility is increased through cross-trained operators;
- An opportunity to test trial modifications to the plant’s control and automation systems and process configurations is provided;
- Development and refinement of operating procedures is facilitated.
An OTS ensures plant operators receive plant specific and realistic hands-on training ahead of plant startup and throughout plant operation.
Integrated approaches to achieve high standards of performance
Achieving a smooth transition from construction to commissioning and startup, followed by ramp-up to full capacity and achievement of the operational performance set out in a project plan, is a challenge for any oil and gas project, but particularly so for large gas liquefaction plants. Lessons need to be learned from previous projects and other operators. Industry best practices need to be established, deployed, and benchmarked.
Preparation for commissioning, startup, and production process operations must be planned in an integrated fashion at an early stage of project planning. Some organizations rigorously implement “flawless start-up” or “perfect plant” methodologies through all project phases through to operations. The integrated implementation of operational-excellence programs before startup is useful in achieving specified design objectives without encountering problems at the commissioning and operations stages.
Central to a flawless startup program is the systematic application of lessons learned from earlier projects and earlier phases of projects as they progress. An extensive “flaw database” aids an integrated approach. This is built up from the experience of an organization or project team, containing lessons learned on all kinds of related projects, which helps to identify potential flaws. It can be complemented and added to by the experience of the contractors, subcontractors, and suppliers involved in the project. Flaws can then be systematically scanned for across the facility components. To be successful this approach needs to be embedded deep into the supply chains of the EPC contractors during construction as a quality assurance measure.
Figure 11 illustrates the functions, management, and reporting activities that require integration. No single team in the plant design, construction, and operational life cycle has control over all factors affecting functional and financial performance of a facility. However, the facility sponsor through its management system needs to ensure their integration and overlap, which will require appropriate information technology and database systems.
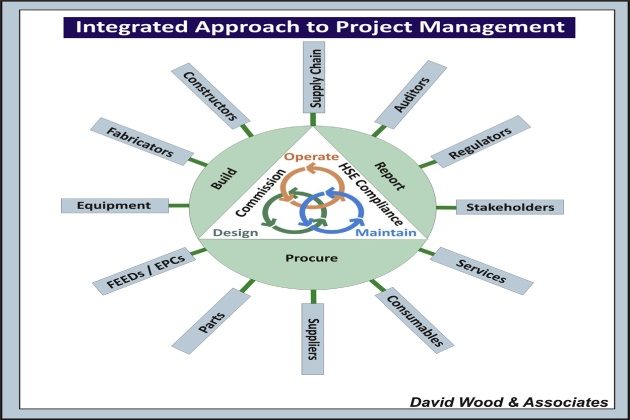
Isolation between the many groups involved in designing, building, operating, and maintaining such facilities wastes time and money, jeopardizes plant safety, and limits the owner-operator’s ability to optimize plant performance.
To prepare for an intended flawless startup and subsequent high-performing plant operations, all project and operations staff need to be trained and provided with tools to enable them to detect and root out defects and potential problems that are often concealed within the process designs.
Recruiting and training quality staff is essential to the success of LNG plant projects and the long-term operations that need to follow on from a project’s development. Because of the breadth and scale of activities in large projects it is important to be able to attract the required numbers of competent staff with relevant experience from recently completed, similar, or related projects. Continuity of core staff is essential and several project team members ideally need to remain in the permanent operating organization well into the operational phase of a new facility. Recruitment of experienced operational staff needs to begin early in project development (i.e., not long after a positive final investment decision (FID) is made). Key operators and technicians need to be recruited, trained, and mobilized to a project site long before the commissioning phase.
An integrated project team can influence long-term project value and return on capital invested in some key ways:
- Define responsibilities, accountabilities, and interfaces across the team at an early stage in the project;
- Measure value and risk across all component parts of a project (using a comprehensive risk register) to focus attention;
- Manage and consult with all project stakeholders with a professional project culture;
- Align the vision of the project objectives across the project team and repeatedly reinforce that vision;
- Focus on how value can be improved and risk reduced at all stages;
- Rigorously and consistently deploy constructability principles to drive project execution.
Conclusions
Detailed, systematic, team-involved plans are the foundation for project success in the LNG industry. Project success means completing all project deliverables on time, within budget, and to a level of quality that is acceptable to the project sponsors and stakeholders. The project manager must keep the project team’s attention focused on achieving these broad goals and the stakeholders aligned to the project objectives. Project risk assessment, mitigation strategies derived from scenario-based risk identifications, and attention to contingency plans for dealing with low-likelihood extreme risk exposures play a key role in the successful design and delivery of LNG projects.
Attention to constructability principles in the planning stages can also reduce the risks of cost, time, and quality problems during the project construction phase. LNG facility construction projects are not complete until the plant, unit, or equipment is placed in service and has demonstrated that it can deliver efficiency levels and uptime availability at or above its design specifications. It may take more than a year after a facility is commissioned and starts operations before acceptable full-capacity performance levels can be established and verified. At that stage the long-term operating team must to an extent live with what a project team has handed to them.